Lost a limb? No Problem!
Andrea Khoury, Nikki Lal, Spencer Levine, Laura Camila Penuela Cardenas
Abstract
Life in the wild is extremely harsh. Major injuries such as the loss of a limb can quickly turn fatal for many animals if they are not protected by other individuals. Since an event like this is extremely dangerous if left unrepaired, many animals across vast phyla have evolved methods to solve this predicament. The solution is limb regeneration! This paper will explain the process of regeneration as seen in the Chordata, Echinodermata, Mollusca and Arthropoda phyla. In specific, the paper analyzes representative species from each phylum. For Chordata, we look at axolotls and zebrafish; for Echinodermata, different starfish species; for Mollusca, members of the Octopoda order; for Arthropoda, cockroaches, and spiders. Further, we briefly extend the concept of limb regeneration beyond the kingdom Animalia and into Plantae. Finally, for those animals who have not evolved such an amazing process, we look at problems related to limb loss and the potential adaptations that are made possible by engineering.
Introduction
Regeneration is an extremely complex and important process for many animals across a wide range of phyla. But what exactly constitutes regeneration? According to Merriam-Webster, regeneration is, by definition, the “renewal or restoration of a body, bodily part or biological system […] after injury or as a normal process,” (“regeneration” | Merriam-Webster, n.d.). In specific, when studying biology, regeneration is viewed as the regeneration of tissues and from there can complexify into larger structures such as organs or limbs. Regeneration is an ongoing and normal process, as seen by exoskeleton molting in spiders (Fig. 1) and snakes, or even shedding fur in domestic animals such as cats and dogs.
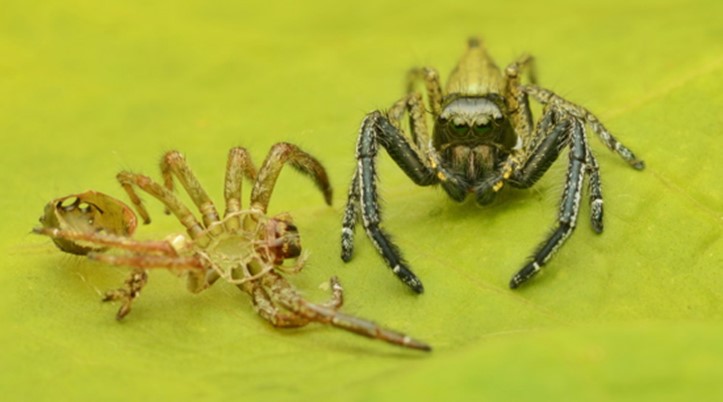
However, in this text, we are more interested in the nonroutine complete regeneration of limbs. In specific, we want to explain the processes that enable such a remarkable process to be coordinated. Many animals, such as dogs, cats, and humans, only generate new limbs during gestation while their cells are undifferentiated (or referred to as stem cells). Perhaps, studying the animals that have evolved this mechanism will lead to new bioengineering innovations so that any animal can benefit from this process. As mentioned in the appendix, we will study the process in the Chordata, Echinodermata, Mollusca and Arthropoda phyla of animals as well as briefly in plants. In specific, we look at axolotls, zebrafish, different starfish species, members of the Octopoda order, cockroaches, spiders, and various plant species.
Before delving into this captivating topic, there are a couple of terms that we need to be familiarized with. There are two types of regeneration: reparative and restorative. Reparative regeneration is when a “multicellular organism has the power only to repair certain damaged cells” (“12th Class Biology Growth, Regeneration and Ageing Repair and Regeneration” | Study Adda, n.d.). In other words, this type of regeneration is limited and is commonly seen in all animals. On the other hand, restorative regeneration is when a “multicellular organism can redevelop the severed body parts or the whole body can be formed from a body segment” (“12th Class Biology Growth, Regeneration and Ageing Repair and Regeneration” | Study Adda, n.d.). Restorative regeneration is the type that we will discuss in detail. Further, there are two mechanisms by which it can occur in animals: epimorphosis and morphallaxis. As T.H. Morgan characterized, epimorphosis is a mechanism where an injured body part is restored via the division/creation of new cells from the surface of the injury. In contrast, morphallaxis is the “reconstruction of an entire animal [or part] from a small fragment by reorganizing existing cells” (“12th Class Biology Growth, Regeneration and Ageing Repair and Regeneration” | Study Adda, n.d.). In this manner, the regenerated portion must grow over time to reach its physiological size. With our foundation of knowledge set, let us meet our specimens of limb regeneration!
Regeneration Across Different Animal Phyla
In this section, we will observe how the process of limb regeneration varies not only across different animal phyla, but also between various animal classes by looking at five examples of species which are known to have the capacity to regenerate their limbs.
Chordata
Amphibia – Axolotl
The Ambystoma mexicanum, more commonly known as axolotl, is an endangered type of Salamander which originated in the lake complex of Xochimilco in Mexico (“Axolotl” | National Geographic, n.d.). This aquatic animal has been the focus of many scientific studies in the past decades for it has the ability of completely regenerating various body parts such as its spinal cord and its limbs at different stages of its life cycle, as seen in Fig. 2.
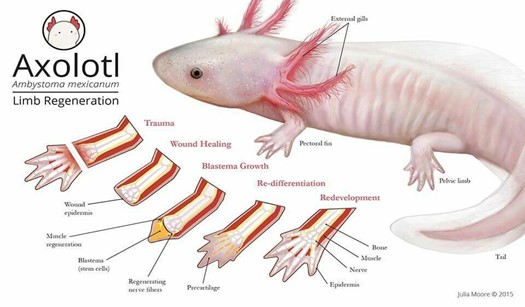
Following limb amputation, the first thing that happens in axolotl limb regeneration is wound healing. At this level, there is fast coagulation at the site of injury succeeded by immune activation and cell death (Tanaka, 2016). After a few hours, the wound epidermis (WE), which is made of keratinocytes – epidermal epithelial cells – that surround the wound, covers the stump. Once the epidermal wound is formed, there is a transient phase of fibrosis followed by the repair of fibrotic tissue, which in the end leads to the restoration of normal skin architecture. The next step is characterized by the formation of the early blastema. After receiving specific nerve signals, “the WE is induced to form the apical epithelial cap (AEC)”: a thick layer set to cover the whole tip of the limb (McCusker et al., 2015). Afterwards, fibroblasts get ‘recruited' and progenitor cells, which may be obtained from stem cells or from a process of dedifferentiation, are activated inside the tissues of the stump. These cells then begin to proliferate underneath the epidermis and accumulate at the tip of the stump thus making up the accumulation blastema (Tanaka, 2016). As the latter has an absence of nerves and blood vessels, it has yet to undergo a development process which is achieved through “coordinated growth, morphogenesis, and differentiation to replace amputated structures” (Stocum, 2017).
The formation of the blastema is essential to the completion and success of axolotl limb regeneration. As a matter of fact, it is the growth and multiplication of the cells making up the blastema that allows them to “[regain] their identity as fully-developed bone or skin cells”, ultimately permitting the re-development of the lost structure (“Axolotl” | National Geographic, n.d.). Furthermore, Kragl et al.'s study consisting of “comprehensively tracking limb tissues marked by an integrated green fluorescent protein (GFP)–transgene in the [Ambystoma mexicanum]” revealed that blastema cells possess two different types of memory of cellular origin: one is lineage-specific and the other is positional. The lineage-specific memory encodes information on limb and parent cell phenotype, therefore limiting cell redifferentiation (Kragl, “Cells keep a memory of their tissue origin during axolotl limb regeneration”). For instance, blastema cells which were obtained from Schwann cells can only re-differentiate as Schwann cells (Fig. 3).
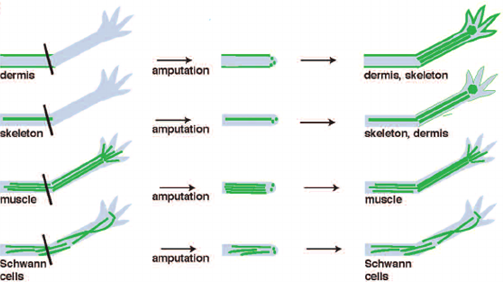
Moreover, the positional memory serves as a reminder to fibroblast-derived blastema cells of where they were originally located with respect to neighboring cells. In the case of blastema cells which do not come from fibroblasts, their position during pattern formation can vary but it is nevertheless regulated by fibroblast-derived cells (Stocum, 2017). It is thanks to these two memory mechanisms that cells making up the blastema know whether they only need to recreate a part or the entirety of the amputated limb. In addition, the mechanisms also indicate to the cells what size the rebuilt structure must be according to the age and size of the animal.
Actinopterygii – Zebrafish
Danio rerio, also known as zebra fish, is a freshwater fish native to South Asia that has great genetic similarity to humans. As a matter of fact, “84 per cent of genes known to be associated with human disease have a zebrafish counterpart” (“Why use the zebrafish in research?” | yourgenome, n.d.). Although this animal has been widely studied to gain a better understanding of gene function, vertebrate development, and diseases like cancer, it has also been carefully looked at for its regenerative capabilities. Indeed, the zebrafish can regenerate its heart, retina, and fins, amongst others (Beffagna, 2019). Here, we will be considering the fins as the ‘limbs' of the Danio rerio and will notably observe how these animals regrow their caudal fin after having suffered an amputation.
In the case of the zebrafish caudal fin, the accumulation of progenitor cells mostly takes place. Interestingly enough, the process of tail fin regeneration of the zebrafish is quite similar to the manner in which axolotls regrow their limbs. In fact, the fish also goes through the steps of wound healing, formation of the WE, creation of the blastema, re-differentiation and redevelopment. However, there are certain considerable differences between the two processes which begin to arise at the step where the formation of the blastema occurs. In the case of the zebrafish caudal fin, the accumulation of progenitor cells mostly takes place in the regions that are distal to the bony rays (Fig. 4).
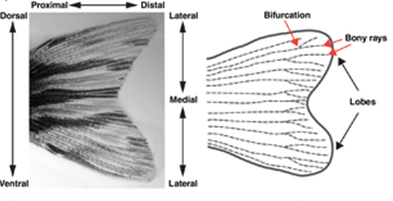
In addition, it seems that progenitor cells tend not to agglomerate in the space that is between the rays. Therefore, there is not a singular large blastema that ‘envelopes' the WE, but rather multiple small blastema that each fin ray forms (Sehring and Weidinger, 2019) (Fig. 5).

On another note, there is a peculiarity about caudal fin restauration that is important to consider and that is allometric scaling. The fin normally undergoes isometric growth. Nevertheless, in the case where it gets amputated, it automatically switches to allometric scaling until the completion of the regenerative process after which it goes back to isometric growth (Sehring and Weidinger, 2019).
What is more, it remains unclear how zebrafish are capable of only regenerating the lost structure as the notion of positional memory that was applied to axolotls cannot quite be used for these fish. This can be explained by the “rather uniform anatomy of the fin rays along the proximodistal axis” (Sehring and Weidinger, 2019). The one thing that has been proven, nonetheless, is that the cells of each bony ray contain positional and length information proper to themselves (Shibata et al., 2018).
Echinodermata
Echinodermata are invertebrate, marine organisms, like Asterias rollestoni and Leptasterias hexactis. A. rollestoni is a starfish native to China and Japan, while the starfish L. hexactis is native to the United States of America. Like salamanders, starfish may readily regenerate their limbs and have also been researched for decades (Kumar and Brockes, 2012). Certain starfish species, such as L. hexactis, have a morphallactic-like process of regenerating their limbs: they regenerate by transforming existing tissue cells and without the presence of blastema (Mladenov et al., 1989). Regeneration in starfish follows similar stages to the regeneration of salamanders. At the moment of amputation, wound healing begins.
Initially, the wound closes at the stump as epidermal cells dedifferentiate to form a protective pre-epidermis at the surface of the wound. This re-epithelialization creates a temporary wound closure while histolysis — the breakdown of cells — and phagocytosis take place to remove the damaged tissue under the epidermal layers (Fan et al., 2011; Mladenov et al., 1989). After this, epidermal cells near the wound interact with dermal cells, forming masses of connective tissue cells and, in cases of epimorphosis, these cells partially differentiate into blastema-like structures of cells. In the case of A. rollestoni, blastema-like structures were observed by staining and magnifying samples of the wound area just four days after amputation (Fan et al., 2011). L. hexactis, on the other hand, only forms masses of connective tissue, while the starfish's muscle cells begin differentiating (Mladenov et al., 1989).
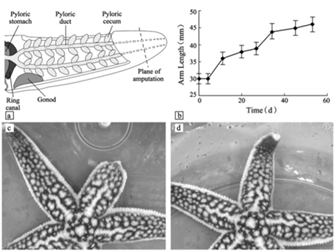
Blastema or none, the cells then continue to differentiate and proliferate, entering the morphogenesis stage of regeneration, where the regenerated arm stub starts to take shape. The growth of the regenerate continues until full length is achieved, which was seen to be around 53 days for the A. rollestoni particularly (Fan et al., 2011). Morphallactic regeneration also lasts about the same amount of time as ephimorphosis, as seen in Fig. 7.

With epimorphic or morphallactic regeneration, as well as being able to grow multiple limbs from one wound, starfish species show great diversity in their regenerative abilities (“The Dividing Starfish” | NZ Marine Studies Centre | YouTube, 2015).
Mollusca
Cephalopod mollusks are capable of regenerating tissues very well. Octopoda, particularly, use their limbs to move, hunt, reproduce, and several other actions; therefore, it is fitting that they have evolved to efficiently close wounds and regenerate full limbs (Shaw et al., 2016). One species of octopus, Octopus vulgaris, is a frequently referenced example of the regeneration of cephalopods. O. vulgaris is capable of not only regenerating its limbs, but also autotomy: self-amputating its own limbs when necessary (Tsang, 2016).

The limb regeneration of cephalopods, demonstrated by O. vulgaris, is again similar to that of starfish. One peculiarity in cephalopod regeneration is the noted presence of acetylcholinesterase (AChE), a glycoprotein, during the arm regeneration stages. AChE is an enzyme whose isoforms can contribute to cell proliferation, cell differentiation, and apoptosis (Tsang, 2016). The levels of this enzyme match up with stages of the regeneration process seen in O. vulgaris.

The first stage of regeneration for O. vulgaris is the formation of blastema and cell proliferation. Mesenchymal cells – stem cells that repair tissue – accumulate at blastema regions as well and, using an enzymatic assay, it is seen that AChE activity is generally low during this early stage (Fossati et al., 2013). There are also hemocytes present; these cells are phagocytes, part of the immune systems of invertebrates. The hemocytes aid in the formation of blastema for the regenerating stub (Imperadore and Fiorito, 2018). As cells proliferate at the arm tip, hemocytes phagocytose debris present at the wound site and as the arm regenerates, all while AChE activity increases steadily. AChE activity spikes around the stage of morphogenesis and myogenesis – the formation of muscular tissue. Although there is no strong understanding of the exact role of AChE in limb regeneration, there is evidence to show it is strongly correlated with the stages of morphogenesis and myogenesis in regeneration (Fossati et al., 2013). Like the other phyla, following morphogenesis is differentiation and reestablishment of the limb structures of the new limb, while cell proliferation continues until completion at the arm tip (Fossati et al., 2013).
The regeneration processes for O. vulgaris take up to 130 days. The fascinating mechanisms involved in Cephalopoda regeneration span the regeneration of nerves, skin, muscles, and appendages. There is still very much about the inner mechanisms and histology of these marine organisms' tissue regeneration that are yet to be found (Imperadore and Fiorito, 2018).
Arthropoda
Regeneration is quite common for arthropods. Many species, such as cockroaches and spiders, may regenerate their limbs, and in other cases, nerves, muscles, and tracheae (Maruzzo et al., 2005). The general mechanism of arthropod regeneration is similar to the general stages of limb regeneration in the aforementioned phyla and, because of this, this section will discuss more about the characteristics of arthropod regeneration than the stages. For appendage regeneration to occur, specifically in arthropods, there must be a detachment of the epithelium from the cuticle near the wound site and the formation of a local blastema (Maruzzo et al., 2005). Regeneration begins with a wound, causing immediate wound healing mechanisms and the formation of blastema. Continuing the histological process of wound healing, cell migration and differentiation takes place to slowly regenerate the limb (Maruzzo and Bortolin, 2013). This is the general basis of cellular regeneration for most arthropod limbs and certain species have their own disparities in the process. Decapod crustaceans, for example, regenerate their limbs outside their epidermis at the wound site, as shown in Fig. 10.
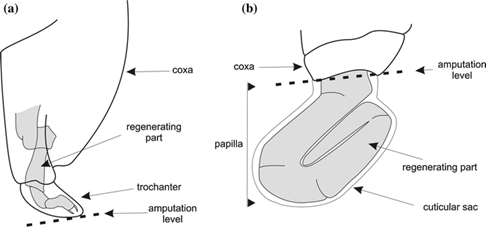
The regeneration occurs in a transparent cuticular sheath known as the papilla (Maruzzo and Bortolin, 2013). This phylum contains such a variety of regeneration abilities, including autotomy and limb regeneration being limited to certain species, that there is a distinction between regenerative potentials between the different taxa of arthropods (Maruzzo and Bortolin, 2013).
Maruzzo and Bortolin expand on four regenerative potentials seen in arthropods, accordingly named absent, poor, good, and very good. Having an absent regenerative potential means that no regeneration will occur. Poor regenerative potential is similar, but in this case, the limb begins to regenerate and does not fully complete its process. Good and very good regenerative potentials differ only by the conditions under which regeneration occurs: specific conditions must be met for a limb to regenerate into a “normal” limb for an arthropod with good regenerative potential, whereas one with a very good potential can regenerate properly under most conditions. In addition to the taxa of the arthropod, the developmental stage of the organism and the limb's degree of amputation also affect the regenerative potential of the limb (Maruzzo and Bortolin, 2013) (Fig. 11).
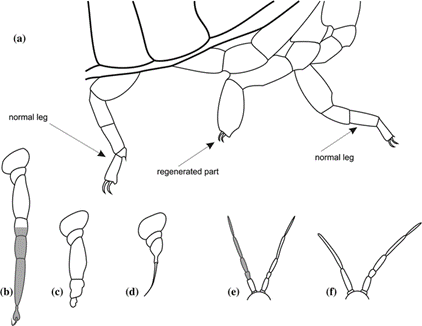
An example of very good regenerative potential is displayed by cockroaches. The regenerated limbs of cockroaches end up structurally normal under most conditions, although, the regenerated limb is not a duplicate of the original. Because interaction between the several layers of tissue is different from the development of limbs, limb regeneration often results in slight discrepancies between the regenerate and the original limb, for example, a different number of segments (Maruzzo and Bortolin, 2013). Another species, the whip spider in the order Amblypygi, always regenerates sections of their forelegs with more segments than their initial forelegs. A direct correlation was made between the body size of the spider and the number of segments it regenerated. This occurs because limbs generated in embryos are sized for younger spiders, so the adult spiders that regenerate limbs often regenerate extended limbs; younger whip spiders regenerate with less segments than adults (Minelli et al., 2010).
Although there are disparities between original and regenerated limbs in several species, this does not affect the overall functionality of the limb (Maruzzo and Bortolin, 2013). As for chordates, echinoderms, and cephalopods, the molecular regeneration of arthropod limbs is much more complex than explored in these pages.
Limits of Limb Regeneration
Although several organisms have the ability to regrow their limbs after amputation of the latter, there is finite number of times during which the process of restoration can take place. Hence, our focus will now be shifted toward some of the things that cause the limitations of limb regeneration and how these restrictions may vary between species.
In general, the capacity that specific animals have to regrow their extremities reduces as the organisms develop. For example, when Xenopus – a type of aquatic frog – are at the tadpole stage, they can restore their limbs if they suffer an amputation, but as they become adults, they lose this ability. Nonetheless, this general observation does not apply to all species. This is the case of axolotls whose capacity of limb regrowth is not affected by ontogenetic development (Dunlap, 2018). Additionally, it is not rare to note that the more a limb is amputated, the more the regeneration process may be hindered. From a study carried out on axolotls in 2017, it was concluded that repetition of limb restoration might cause a higher risk of wrongful expression of a factor called amphiregulin. Given the case where the latter is not expressed correctly, the whole limb regrowth process is completely stopped (Bryant, 2017) (Fig. 12).
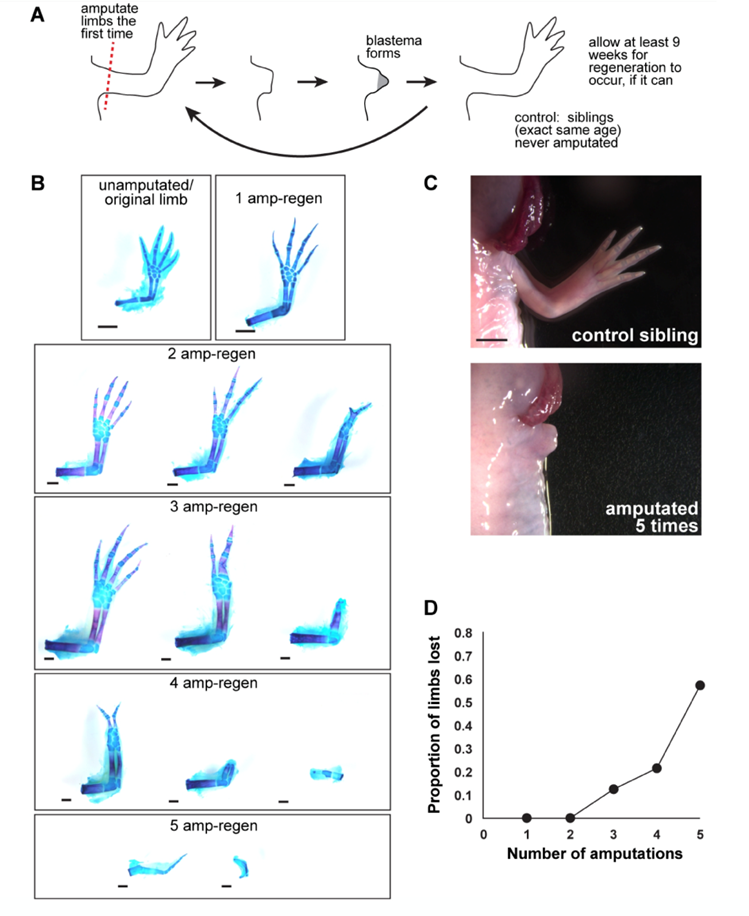
Delving into some more particular limitations, we found three common ones. The first that we will look at is the abundance of progenitor cells. As was previously discussed and demonstrated, these cells play a key role in the mechanism of limb restoration. Thus, it can be theorized that the amount of progenitor cells present “reflects the regenerative capacity” (Zhao et al., 2016). It was remarked that axolotls and zebrafish do not necessarily require a large amount of these cells to keep on restoring their limbs, however. This is related to the second limit we came across: the differentiation potential. As a matter of fact, Ambystoma mexicanum and Danio rerio have a high potential of cell differentiation which allows them to produce new progenitor cells (Zhao et al., 2016). The last limit we noticed was the concept of presence of regeneration genes. Essentially, researchers have hypothesized that animals may have some genes that are proper to regeneration and that are either expressed or not depending on the limb regrowth ability of organisms. A study conducted on newts – a type of salamander – by Garza-Garcia et al. identified that a gene called Prod1 “is expressed in the blastema and essential for patterning and growth during the salamander limb regeneration” (Garza-Garcia et al., 2010; Zhao et al., 2016). These researchers equally discovered that the Prod1 gene can only be found in salamanders. Therefore, these findings would support the hypothesis mentioned above.
Regeneration in Plants
The research into the mechanisms of regeneration and the molecules involved continues today and is not only limited to the kingdom Animalia which we have covered so far. In this section, we will briefly discuss the amazing regenerative properties of plants. After all, the branches of trees are referred to as limbs and even the roots serve a quasi-limb function by anchoring the plant into the ground in the same way animals use legs to stand in one spot.
In our everyday lives, it is actually really easy to see the regeneration of plants. Branches and leaves are always in the process of regenerating. If you wait a long enough period of time, you can notice that any branch that has fallen for any reason will reappear. The amount of time it will take depends on each species.
However, it would be technically incorrect to say any branch regrows. In fact, it is accurate to say that a new branch is formed from the location where the old branch used to be. This feat is accomplished by latent or dormant buds (Fig. 13).
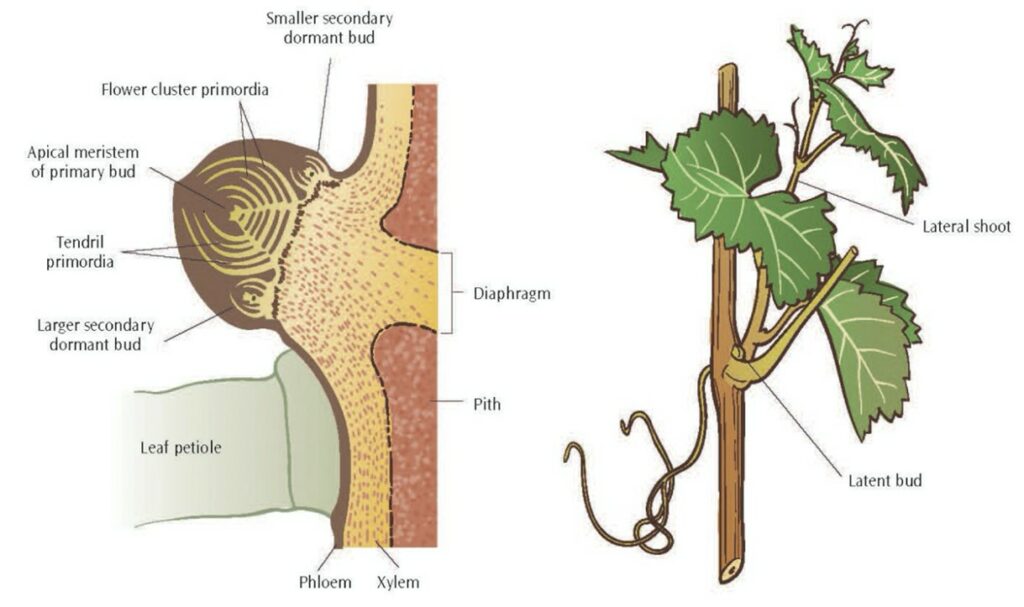
These buds are dormant and typically concealed; in the event of an amputation of a branch, they will be responsible for generating the shoots to replace what was lost with the same mechanism for the development of normal buds.
Moreover, and perhaps more interestingly for biologists and bioengineers, plant cells may undergo cell dedifferentiation just like some of the animals discussed earlier in this paper! In vitro experiments have demonstrated the ability of explanted living plant tissues to dedifferentiate through generations of mitosis, and re-differentiate to form a complete, fertile plant with all of its limbs. In Fig. 14, we see one such experiment where isolated, fully differentiated carrot root cells were explanted into a culture beaker and underwent the aforementioned process to develop “all of the organs of a mature carrot plant” (Kimball, 2020).

All of this section is designed to broaden our scope a little and consider that regeneration is not a process limited to one kingdom of life. Now, we shift our focus back to animals, under a different context.
Coping Without Regeneration Mechanisms
Difficulty of adaptation for animals who cannot regenerate – Example of the Alligator
One of the worst disadvantages an animal can have is lacking a limb. Limbs are extremely useful to survive in a natural environment. In nature, the sole purposes of life are to survive and reproduce. These two fundamental parts of animals' lives are practically impossible to take place when lacking a limb. Alligators for example, have four limbs and a tail. Similar to the elephant's trunk (Khoury et al., 2020), the alligator's tail can be understood as a fully-fledged limb since it is vital for walking around, swimming and defending its beholder. It even represents 28 % of total body mass! The role of hindlimbs and the tail in body mass support are similarly computed in the calculation of body mass (Willey et al., 2004). Thanks to this functional limb, the alligator can hydroplane on the surface of the water. Moreover, the tail is critical for maintaining its balance and buoyancy in the water. An alligator's tail comprises half of the animal's body length and is powerful and muscular. Furthermore, this reptile's muscular tail allows it to jump as high as 5 feet above the water. Losing this precious limb imperils survival in its natural habitat (Potter, 2019).
Mr. Stubbs is an alligator who got his tail eaten by another alligator when he was 13 years old (Fig. 15).
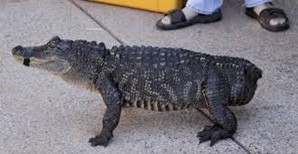
This unique occasion caught the attention of engineers who wanted to try something revolutionary. First, engineers and researchers dressed it with a prosthetic tail made from the cast of a similar-sized dead alligator's appendage (Hertzberg, 2018). They built many expensive prosthetics which did not actually correspond to the animal's anatomy, center of mass and unique buoyancy. As a result, Mr. Stubbs could not bear the prosthetic and outgrew it as he matured and did the same for many other subsequent artificial tails (“Amazing Animals With PROSTHETIC LIMBS” | Origins Explained | YouTube, 2018) (Fig. 16).
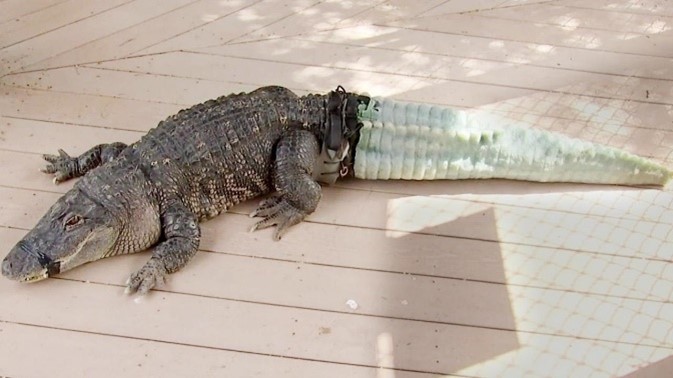
After 5 years of technological inventions, 3D Scanning and printing became a viable method to create artificial limbs and body parts. Engineers and researchers were able to build a perfectly sized and perfectly weighted tail. This new device was measured to the millimeter. As a matter of fact, features even bellow the millimeter scale are visible and usable! The tail would not burden its balance and swimming motions anymore. Engineers used an Artec3D Scanner (“An Alligator Named Mr. Stubbs Now Sports A 3D-Printed Tail” | WBUR, 2018) (Fig. 17).
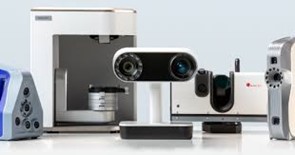
The material they used to create this 3D Printed tail is called Dragon Skin, and they use it again in human prosthetics. It could interact with skin for very long period of time and not cause irritation or uncomfortable feelings (“An Alligator Named Mr. Stubbs Now Sports A 3D-Printed Tail” | WBUR, 2018). Several weeks after putting on his new tail, Mr. Stubbs happened to tail-slap a volunteer at the rescue facility. This is at first shocking for the helper, but at the same time, really promising. The reptile felt comfortable enough with his new limb that he was able to use it in a natural fashion (Hertzberg, 2018).
Functional adaptation of humans
Unlike the Alligator and many other animals in the wild, humans are not considered as prey to other animals when lacking a limb. As a matter of fact, a human can lose a limb and still live a full life. Multiple factors can lead to a limb amputation in humans such as congenital limb difference/absence, cancer, trauma, etc. In the United States, there are 2.1 million people living with limb loss, and there are about 185 000 amputations each year. This means humans need to adapt to a natural environment which is not suitable to their peculiarities (There are physiologic responses and reserves in the body following these losses) (“Limb Amputation Ground Rounds: Cardiopulmonary Adaptations, Pharmacokinetics, and Phantom Limb Pain” | VUMC Department of Anesthesiology | YouTube, 2020).
For disabled people to continue making music and playing instruments, an organization called OHMI (One-Handed Musical Instrument Trust) was created by a 23-year-old musician born without arms. He uses his feet to eat, dress up, write, and wanted to pursue a musical career as well. His handicap did not stop him from realizing his dream. This organization helps fund the development of adapted or specially designed instruments for musician with one hand and other limb differences (Tracey, 2014) (Fig. 18).

Like most woodwind instruments, the diatonic scale is played by opening a row of holes in the tube wall. Side holes may then be added for full chromaticism and trills. Maarten's saxophones use ‘reverse action' on the upper keys to close the holes when at rest, together with carefully positioned extra keys that then allow the entire range of the instrument to be played with one hand (“Woodwind Instruments” | OHMI, n.d.).
Regenerative powder
Regrowing extremities of animals artificially for animals who cannot do it naturally felt surreal for many decades. It was no later than 2011 when an extra-cellular matrix (ECM) powder from pigs' bladders appeared to be effective at regrowing a finger. Nerves, tissues, blood vessels, skin regrew in 4 weeks with the help of this powder (“Stem Cell Powder Regrows Finger” | StemcellTv | YouTube, 2011). The cells found in the pig's organ possess remarkable regenerative properties. Integrated inside the pig's structure, there is a series of powerful molecular signaling agents that suppress the scarring response and summon stem cells to create new tissue (Piore, 2017).
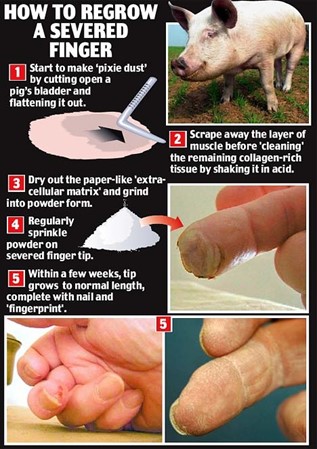
Researchers scrap the cells from pig's bladders, dry out the tissue and then form a powder. Instead of going into a whole healing process and ending up with a scar, spreading the ECM powder on the wound stimulates the cells to regrow (“Stem Cell Powder Regrows Finger” | StemcellTv | YouTube, 2011). The powder is mostly collagen and variety of substances, without any actual pig cells. Spreading this powder on the wound forms microscopic scaffolding for human cells to occupy. Then it emits chemical signals to encourage those cells to regenerate tissues (Batty, 2008).
Conclusion
In this research paper, we explored the process of limb regeneration for animals of different phyla and the limits of their regeneration. We then examined reasons behind the finiteness of the regeneration process in animals, regeneration in plants, and possible coping mechanisms aside from the regeneration of amputated limbs.
From the analysis of five animal species, we noticed that member regeneration can occur in two different manners: either by epimorphosis or by morphallaxis. Epimorphosis begins with the proliferation of somatic/progenitor cells, followed by their dedifferentiation, and the formation of a blastema. Subsequently is the re-differentiation and morphogenesis of the new structure. This type of restoration process was observed in the phylum Chordata, Mollusca, and Arthropoda. Alternatively, morphallaxis consists of the transformation and re-patterning of existing tissues, particularly without the formation of blastema. This mechanism was seen in species of the phylum Echinodermata. Among the regeneration mechanisms of the species discussed, we touched on the topics of cellular memory, progenitor cell behavior in zebrafish, the role of AChE in cephalopod regeneration, and regenerative potentials in arthropods.
The limits of animal regeneration differ by species and is often the cause of ontogenetic development, with axolotls being among the exceptions. Other limitations include the number of times an organism has regenerated the limb, the amount of progenitor cells present, the regenerative potential of the cells, and the hypothesized presence of regeneration genes. As for plants, there are far fewer limitations, as they contain cells responsible for the regrowth of branches, their “limbs”, that undergo cellular dedifferentiation like animal cells.
Finally, we discussed some coping mechanisms for animals without the ability to regenerate amputated limbs. In the case of the tailless alligator, which is extremely vulnerable to predators, bioengineers created a perfectly sized and weighted tail using a 3D Printer. For humans, some functional adaptations are modified musical instruments which allow people lacking a limb to still make and play music, and an extra-cellular matrix powder from pig's bladder that has the ability to regrow extremities in animals lacking regenerative properties. The understanding of cellular regenerative mechanisms is still vague in comparison to all that we do not know, but as the science continues to develop, we continue to engineer incredible solutions to various biological needs regarding regeneration.
Appendix
“Alligator Named Mr. Stubbs Gets a Prosthetic Tail.”, 11 July 2018, Inside Edition, YouTube, https://www.youtube.com/watch?v=qJ0S-VMN1N4.
“Amazing Animals With PROSTHETIC LIMBS.”,1 November 2018, Origins Explained, YouTube, https://youtu.be/Iamyjl7MDic .
“Limb Amputation Ground Rounds: Cardiopulmonary Adaptations, Pharmacokinetics, and Phantom Limb Pain.”, 5 June 2020, VUMC Department Of Anesthesiology, YouTube, https://youtu.be/aaLKRpFEcQw.
“Stem Cell Powder Regrows Finger.”, 2 November 2011, StemcellTv, YouTube, https://youtu.be/NEnLK0oJCa8.
“The Dividing Starfish.”, 19 April 2015, NZ Marine Studies Centre, YouTube, https://www.youtube.com/watch?v=AaN6uRvfPLY&t=133s.
References
3D, A. (n.d.). Artec 3D technologies to be showcased at the Free 3D Scanning Open House at Indicate Technologies. Retrieved from https://www.artec3d.com/events/indicate-technologies-open-house-2020
Adda, S. (n.d.). 12th Class Biology Organisms and population Repair and Regeneration. Retrieved from https://www.studyadda.com/index.php?/notes/12th-class/biology/growth-regeneration-and-ageing/repair-and-regeneration/9771
Batty, D. (2018). Regrown finger is ‘junk science'. Retrieved from https://www.theguardian.com/science/2008/may/01/finger.claim
Beffagna, G. (2019). Zebrafish as a Smart Model to Understand Regeneration After Heart Injury: How Fish Could Help Humans. Front Cardiovasc Med, 6, 107. doi:10.3389/fcvm.2019.00107
Bryant, D. M. (2017). Insights into the mechanisms and limitations of axolotl limb regeneration. (Doctoral Dissertation ). Harvard University Retrieved from http://nrs.harvard.edu/urn-3:HUL.InstRepos:42061474
Dictionary, M.-W. (Ed.) (n.d.). regeneration. In. merriam-webster.com: Merriam-Webster. Retrieved from https://www.merriam-webster.com/dictionary/regeneration
Dunlap, G. (2018). Regeneration: What the axolotl can teach us about regrowing human limbs. Retrieved from https://sitn.hms.harvard.edu/flash/2018/regeneration-axolotl-can-teach-us-regrowing-human-limbs/
Fan, T., Fan, X., Du, Y., Sun, W., Zhang, S., & Li, J. (2011). Patterns and cellular mechanisms of arm regeneration in adult starfish Asterias rollestoni bell. Journal of Ocean University of China, 10(3), 255. doi:10.1007/s11802-011-1837-y
Fossati, S. M., Carella, F., De Vico, G., Benfenati, F., & Zullo, L. (2013). Octopus arm regeneration: Role of acetylcholinesterase during morphological modification. Cephalopod Biology a special issue compiled under the auspices of no-profit research organization CephRes, 447, 93-99. doi:10.1016/j.jembe.2013.02.015
Garza-Garcia, A. A., Driscoll, P. C., & Brockes, J. P. (2010). Evidence for the Local Evolution of Mechanisms Underlying Limb Regeneration in Salamanders. Integrative and Comparative Biology, 50(4), 528-535. doi:10.1093/icb/icq022
Geographic, N. (n.d.). Axolotl Retrieved from https://www.nationalgeographic.com/animals/amphibians/facts/axolotl
Growers, L. W. (n.d.). Important Structures & Features of Grapevines. Retrieved from https://www.lodigrowers.com/important-structures-features-of-grapevines/
Herzberg, R. (2018). How 3D Printing Helped Mr. Stubbs, The Tailless Alligator. Retrieved from https://www.nationalgeographic.com/animals/article/alligator-tailless-mr-stubbs-prosthetic-tail-news
imgur. (2015). Mr. Stubbs: The World's First Alligator With A Prosthetic Tail. In. imgur. Retrieved from https://imgur.com/gallery/2QDmJ/comment/548500893
Imperadore, P., & Fiorito, G. (2018). Cephalopod Tissue Regeneration: Consolidating Over a Century of Knowledge. Frontiers in Physiology, 9, 593-593. doi:10.3389/fphys.2018.00593
Khoury, A., Lal, N., Levine, S., & Penuela Cardenas, L. C. (2020). A Ton of Mechanical Advantages: Analyzing the Remarkable Structure of Elephant Limbs. Bioengineering McGill University. Montreal, Canada. https://bioengineering.hyperbook.mcgill.ca/a-tonne-of-mechanical-advantages-analyzing-the-remarkable-structure-of-elephant-limbs-limbs-1/
Kimball, J. W. (2020). Regeneration. In BIOL3400 Developmental Biology. Retrieved from https://bio.libretexts.org/@go/page/27170
Kragl, M., Knapp, D., Nacu, E., Khattak, S., Maden, M., Epperlein, H. H., & Tanaka, E. M. (2009). Cells keep a memory of their tissue origin during axolotl limb regeneration. Nature, 460(7251), 60-65. doi:10.1038/nature08152
Kragl, M., Knapp, D., Nacu, E., Khattak, S., Schnapp, E., Epperlein, H. H., & Tanaka, E. M. (2008). Novel insights into the flexibility of cell and positional identity during urodele limb regeneration. Cold Spring Harbor Symposia on Quantitative Biology, 73, 583-592. doi:10.1101/sqb.2008.73.034
Kumar, A., & Brockes, J. P. (2012). Nerve dependence in tissue, organ, and appendage regeneration. Trends in Neurosciences, 35(11), 691-699. doi:10.1016/j.tins.2012.08.003
Maruzzo, D., Bonato, L., Brena, C., Fusco, G., & Minelli, A. (2005). Appendage loss and regeneration in arthropods. In (pp. 215-245).
Maruzzo, D., & Bortolin, F. (2013). Arthropod Regeneration. In A. Minelli, G. Boxshall, & G. Fusco (Eds.), Arthropod Biology and Evolution: Molecules, Development, Morphology (pp. 149-169). Berlin, Heidelberg: Springer Berlin Heidelberg. doi: https://doi.org/10.1007/978-3-642-36160-9_7
McCusker, C., Bryant, S. V., & Gardiner, D. M. (2015). The axolotl limb blastema: cellular and molecular mechanisms driving blastema formation and limb regeneration in tetrapods. Regeneration, 2(2), 54-71. doi:https://doi.org/10.1002/reg2.32
Minelli, A., Maruzzo, D., & Fusco, G. (2010). Multi-scale relationships between numbers and size in the evolution of arthropod body features. Arthropod Structure and Development, 39(6), 468-477. doi:10.1016/j.asd.2010.06.002
Mladenov, P. V., Bisgrove, B., Asotra, S., & Burke, R. D. (1989). Mechanisms of arm-tip regeneration in the sea star, Leptasterias hexactis. Roux's Archives of Developmental Biology, 198(1), 19-28. doi:10.1007/BF00376366
Moore, J. (2015). Axolotl Limb Regeneration. In. Pinterest. Retrieved from https://www.pinterest.com/pin/558868634996036799/
OHMI. (n.d.). Woodwind Instruments. Retrieved from https://www.ohmi.org.uk/woodwind.html
Piore, A. (2017). Revisited: The Regenerative Power of Pig Guts. Retrieved from https://www.discovermagazine.com/health/revisited-the-regenerative-power-of-pig-guts
Potter, E. (2019). Parts of an Alligator Body. Retrieved from https://sciencing.com/parts-alligator-body-6909863.html
Ramel, G. (n.d.). Spider Ecology: Taking A Look At Molting, Mimicry & More. Retrieved from https://www.earthlife.net/chelicerata/s-ecology.html
Saladin, K. (2019). Is powdered pig's bladder commonly used in reparative medicine? In. Quora. Retrieved from https://www.quora.com/Is-powdered-pig-s-bladder-commonly-used-in-reparative-medicine
Sehring, I. M., & Weidinger, G. (2020). Recent advancements in understanding fin regeneration in zebrafish. WIREs Developmental Biology, 9(1), e367. doi:https://doi.org/10.1002/wdev.367
Shaw, T. J., Osborne, M., Ponte, G., Fiorito, G., & Andrews, P. L. R. (2016). Mechanisms of wound closure following acute arm injury in Octopus vulgaris. Zoological Letters, 2, 8-8. doi:10.1186/s40851-016-0044-5
Shibata, E., Liu, Z., Kawasaki, T., Sakai, N., & Kawakami, A. (2018). Robust and local positional information within a fin ray directs fin length during zebrafish regeneration. Dev Growth Differ, 60(6), 354-364. doi:10.1111/dgd.12558
Stocum, D. L. (2017). Mechanisms of urodele limb regeneration. Regeneration (Oxf), 4(4), 159-200. doi:10.1002/reg2.92
Tanaka, E. M. (2016). The Molecular and Cellular Choreography of Appendage Regeneration. Cell, 165(7), 1598-1608. doi:10.1016/j.cell.2016.05.038
Tracey, E. (2014). The man who plays French horn with his toes. Retrieved from https://www.bbc.com/news/blogs-ouch-28541455
Tsang, A. (2016). Tissue Regeneration Protein In Octopus And Humans?. Retrieved from https://biol312.opened.ca/tissue-regeneration-protein-in-octopus-and-humans/
WBUR. (2018). An Alligator Named Mr. Stubbs Now Sports A 3D-Printed Tail. Retrieved from https://www.wbur.org/hereandnow/2018/08/17/alligator-3d-printed-tail
Willey, J. S., Biknevicius, A. R., Reilly, S. M., & Earls, K. D. (2004). The tale of the tail: limb function and locomotor mechanics in Alligator mississippiensis. Journal of Experimental Biology, 207(Pt 3), 553-563. doi:10.1242/jeb.00774
yourgenome. (n.d.). Why use the zebrafish in research? Retrieved from https://www.yourgenome.org/facts/why-use-the-zebrafish-in-research Zhao, A., Qin, H., & Fu, X. (2016). What Determines the Regenerative Capacity in Animals? Bioscience, 66(9), 735-746. doi:10.1093/biosci/biw079