Rodent Adaptations to Ever-growing Teeth
Taegan Hallahan, Emma Martin, Marion Vandewynckele-Bossut, Mianchen Wang
Abstract
Rodents have ever-growing incisors as a result of their chew-intensive diets. The potential consequences of this growth are numerous and can cause serious diseases for these animals. To avoid these outcomes, the structures and mechanisms involved in the function of nutrition must adapt to this trait. Therefore, the incisors are continually eroded and shortened due to the foods they consume and the constant gnawing of rough items. Furthermore, the structure of their teeth and jaw muscles have adapted to their ever-growing teeth. Those adaptations will be examined and discussed in the paper.
Introduction
Every species has a specific diet adapted to its energetic needs and availability of environmental resources. This diet is responsible for adaptations in the teeth, mandibular system, jaw muscles, digestive system, etc. of a species such that the breaking down and absorption of the food is optimized. Fig. 1 illustrates to which extent a species' set of teeth can adapt to its diet.
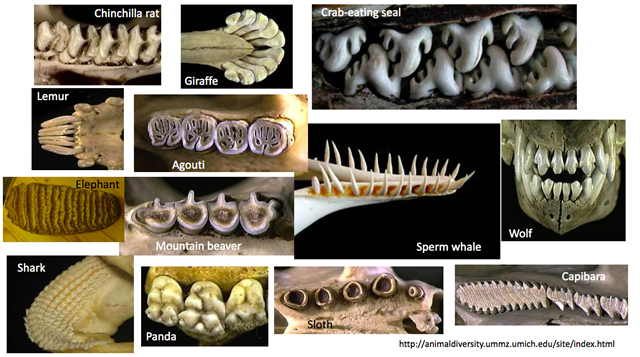
In the area of tooth adaptations to diet, rodents are well-known for their ever-growing incisors. The self-sharpening nature of rodent incisors makes them incredibly versatile beyond simply chewing grain (Druzinsky, 2015). Depending on the species, cutting wood, breaking through the tough skin of prey or tough fruit are use cases for the incisors of a rodent. In a healthy rodent, incisors have the appearance shown in Fig. 2. The incisors are kept sharp and at a length that allows them to grate both upper (Fig. 2b) and lower (Fig. 2d) incisors, by lateral mandibular movement. Those ever-growing teeth of theirs allows them to use arduously their teeth by chewing and gnawing on hard objects (Druzinsky, 2015).
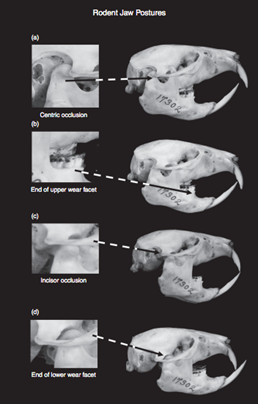
The different mastication modes are an adaptation to these specific diets. While the rodents are known for their gnawing which is defined as “to bite something persistently to injure it or remove portions of it; to wear away by continued biting or nibbling”, other species such as herbivores grind their food “to reduce to powder or small fragments by friction”, or shear to cut sharp (“shear” | Merriam-Webster Dictionary, n.d.).
Dangers of Ever-growing Incisors
Those ever-growing incisors, although essential for their nutrition and lifestyle, are a double-edge sword for their health. Indeed, they are essential for their diet but can also cause serious health problems in the case of overgrown incisors. The whole challenge of a rodent is to wear off its incisors at the same rate as incisor eruption. If it is not the case, dental diseases and malnutrition can occur.
A common dental disease in rodents is anorexia, with symptoms causing oral discomfort, leading the affected animal to appetite loss (Capello, 2008). In severe cases, the rodent may not eat at all. The diet of the animal may also change, since the rodent will prefer softer foods over harder ones, even if the shift may come with a nutritional balance change (Capello, 2008). The causes of dental disease tend not to be cavities since the teeth constantly grow and are replaced. Instead, the constant growth of the incisors, and cheek teeth, depending on the rodent, tend to be the cause. The alignment of upper and lower teeth is key here, with misalignment referred to as ‘malocclusion', which leads to an uneven wearing of the teeth. Molars grow long with sharp points. With movement and increasing length, these molars damage the cheek and tongue of the rodent. Incisors become very long and curl like tusks inwards, growing into the opposing jaw, sometimes being purposely broken off by the affected rodent (Capello, 2008). Once symptoms occur weight loss is rapid.
The causes for malocclusion vary. Already poor nutrition or an unbalanced diet can cause a shift in the root of one or more incisors, or even weaken the jawbone if lacking in calcium, misaligning the teeth as the force of chewing is applied. A genetic predisposition may cause teeth to grow misaligned naturally as well (Osofsky and Verstraete, 2006). These are catastrophic issues that, once present, are usually irreversible and pose a grim outlook for the rodent in question.
In chinchillas and guinea pigs, another dental risk is root elongation (Osofsky and Verstraete, 2006). If the rodent does not chew enough rough items, food will not have been chewed enough before swallowing to provide sufficient wear to the premolars and this can cause the roots of those teeth to extend. As the roots balloon in size, they appear as lumps on the jaw (Osofsky and Verstraete, 2006). If occurring on the upper jaw, these lumps can push up behind the eyes and cause sight problems. In these situations, the crowns of the premolars are usually extended. The growth of the roots is permanent, with intense pain causing a massive drop in appetite, further exacerbating the issues (Osofsky and Verstraete, 2006).

Causal Relationship Between Diet, Morphology, and Bite Force
To avoid these grim outlooks, proper diet and structural adaptations are in order. Let us first take a look at diet, which regulates bite force through changes in morphology, and explore the causal relationship between diet, morphology and bite force.
Among rodents, herbivores, granivores/frugivores, insectivores, and omnivores are different in morphology including the shape and size of their incisors, masseter muscle configuration, and skulls (Freeman and Lemen, 2008). Besides, according to the research from Maestri et al. (2016, p. 2202), rodents — like squirrels — that use their incisors and feed on a high portion of hard food tend to have stronger bite force at their incisors; whereas rodents —like guinea pigs — that use their molars and feed on a high portion of soft food are expected to have stronger bite force at their molars. Hence, it is possible to see that rodents' morphology, diet, and bite force are interrelated (Maestri et al., 2016).
To determine the causal relationships between diet, morphology, and bite force, the researchers built six different models based on six different hypotheses of their relationships and used phylogenetic path analysis (Fig. 4). They found out Path A is the best representative which shows that it is the diet that alters bite force indirectly in rodents by directly influencing the muscle configuration and skull characteristics, and not the other way around. The changes in morphology will then affect their bite force (Maestri et al., 2016).
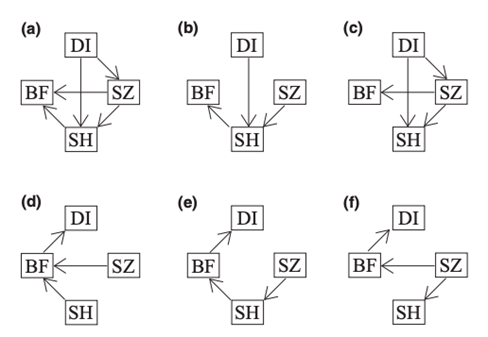
Diet adaptation affects morphology via natural selection (Maestri et al., 2016). During the process of evolution, as food items that rodents used to feed on change in abundance or are not available anymore, to survive, rodents are forced to adjust their bite force by changing their morphology. Those rodents that are not equipped with suitable structure and sufficient bite force will then be penalized and become extinct eventually (Maestri et al., 2016).
Changes in masseter muscle configurations and skull shape will alter and influence the bite force. Based on their masseter muscle arrangement and configuration, rodents are divided into three different morphotypes (Cox et al., 2012) (Fig. 5). Scriuromorph condition involves an extension of the large masseter lateralis muscle onto the rostrum. Compared to other rodents, due to the extensions of the masseter lateralis muscle, scriuromorphous rodents normally have greater gnawing abilities and stronger bite, so it is more effective for them to feed with their incisors (Cox et al., 2012). Squirrels and beavers are characterized by this condition and they mainly feed on hard food like nuts and seeds. In hystricomorph condition, the masseter medialis gets enlarged, passes through the infraorbital foramen and inserts onto the rostrum (Cox et al., 2012). Springhare and jerboas and characterized by this condition which allows them to feed on vegetation using their molars effectively (Maestri et al., 2016). Finally, the myomorph condition combines the sciuromorph and hystricomorph conditions. It involves the extensions of both masseter medialis and lateralis on to the rostrum (Cox et al., 2012). Rats and mice are characterized by myomorphous conditions, and because of their generalist phenotype, they are highly successful species around the world and occupy a large portion of the rodent species (Maestri et al., 2016). Since rats and mice can feed on both their incisors and their molars, there is no trade-off relationship between gnawing and chewing, the two feeding modes. They can feed on seeds, insects, and fruits. Therefore, as generalists, they have omnivorous diets and may survive and thrive in a wider variety of environmental conditions (Maestri et al., 2016).
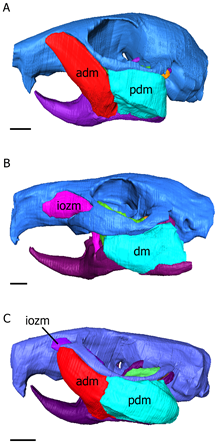
Rodents such as insectivores that have a slender and elongated skull and mandible will have a weaker bite since their elongated skull shape will generate a longer lever arm (Becerra et al., 2011). Because shorter jaws have a shorter lever arm and can produce greater bite force, and wider and tall skulls can contain a higher volume of muscles, rodents that have shorter, but wider and taller skulls will have the greatest bite force in general cases (Maestri et al., 2016).
With suitable structures and adequate bite force, rodents are capable of grinding their incisors on hard food to maintain their dental health and prevent incisors overgrowth and development of malocclusion (Froberg-Fejko, 2014). However, rodent species also have special teeth and jaw adaptations to ensure this strong bite force and the continuous grinding of teeth is not a liability to their dental health. In the next few sections, we will explore these adaptations, starting by the structure and tissue of the teeth.
Anatomy of Rodent Teeth and Comparison to Human Teeth
To understand the functioning of ever-growing teeth, let us first look at the basic anatomy of a normal tooth. The part of the tooth which emerges from the gum is called the crown, and the inside part is called the root. Typically, teeth have the three following layers of tissue, ordered from the innermost to the outermost layer: pulp, dentine and enamel. The pulp is the innermost layer where we find the blood vessels and nerves. It thins as it gets closer to the root-end of the tooth, until it forms what is called a root canal. Dentin is a harder, mineralized layer covered by enamel, the third layer. The enamel is much more mineralized, hence solid, than dentin. In fact, it is only composed of about 4 % of organic material (Myers et al., 2020), which makes it so tough.
Solely in tooth structure, rodents have many adaptations that allow them to use their tooth ardently, like they are required, with minimal corrosion. For instance, a rodent's enamel is particularly hard compared to that of other mammals. While most mammals have teeth classified as a 5 on Mohs' Hardness Scale (a scale to measure the hardness of minerals), rodents' enamel is a 5.5 on Mohs' Hardness Scale (“Rat Teeth” | Rat Behavior, 2004).
Let us take a look at the composition of rodent enamel to account for its toughness. Enamel is mainly composed of inorganic mineralized material that makes up 95 % of mature enamel (“Enamel: Composition, Formation, and Structure” | Pocketdentistry, 2015).
Undoubtedly, there are some organic components which are mainly proteins, namely amelogenin and ameloblastin, to help regulate mineralization. Nonetheless, the mature tissue remains acellular. Indeed, only bone cells, called ameloblasts, exist in developing enamel in the upper portion of the crown so that it is not affected by the tooth grinding of the rodents (Pugach and Gibson, 2014).
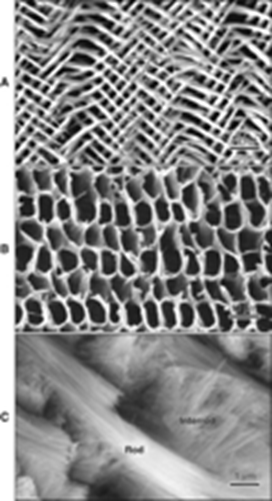
The mineral content is made up of an intertwined and resilient network of rods and interrod. These fundamental units of enamel are composed of packed carbonate apatite crystals. Apatite is a name given for hexagonal phosphate crystals (Themes, 2015). Carbonate apatite is an apatite crystal in which one of the phosphate groups was replaced by a carbonate group, either CO3OH or CO3F. As the enamel matures, these crystals grow and expand until they press against each other such that, in mature enamel, they do not exhibit a hexagonal shape anymore (“Enamel: Composition, Formation, and Structure” | Pocketdentistry, 2015). The crystals that form ribbonlike structures are arranged in rods and interrod that comprises enamel. The figure at the left displays these components where we can identify the rods (Fig. 6a), the interrod (Fig. 6b) and the structure resulting from the intertwining of these two constituents (Fig. 6c) (“Enamel: Composition, Formation, and Structure” | Pocketdentistry, 2015). The great mineral concentration as well as this compact arrangement justifies enamel's resistance to mechanical forces that can be applied to it. It also displays a certain degree of brittleness so that an old layer of enamel can be replaced by a newer, tougher one (“Enamel: Composition, Formation, and Structure” | Pocketdentistry, 2015).
This distinguishable tissue hardness and level of mineralization are beneficial for rodents but they are what makes it extremely arduous for scientists to examine in depth enamel composition. This is why it is still a subject full of speculations and hypotheses. For example, we recognize the existence of rodent-specific components of incisors enamel such as an Fe-rich surface layer and an Fe-rich pigmented transition zone, as well as unusually high levels of Mg and Ca, but are not clear as to what their distinct roles are (Rinaldi and Cole, 2004). However, we recognize the existence of iron substitution in rodent enamel, which makes it even harder and is partially responsible for this intense yellowish color in rodent's incisors (Srot et al., 2017).
Rodents also have special roots that allow them to grow their teeth continuously. In brachidontes (human teeth), the root canal eventually closes off in the mature tooth so that its growth is stopped. In hypsodont (rodent teeth), however, the root canal never closes off, allowing continuous growth of the tooth. We call these kinds of teeth, which rodents are equipped with, “rootless teeth”. The main pre-requisite for good rodent dental health is for the rodent to wear its incisors at approximately the same rate as incisor eruption. For reference, this rate ranges from 0.74 to 1.06 mm a day in beaver teeth (Rinaldi and Cole, 2004)!
This fairly large rate of tooth eruption makes it necessary for them to have appropriate adaptations that allow them to grind their teeth efficiently, while keeping them sharp.
As another adaptation to their specific diet and use of their teeth, rodents have an increased tooth movement allowed because of the additional group of collagen fibers within the periodontal ligament. The periodontal ligament is a group of connective tissues that is responsible for attaching the tooth to the bone within it sits (Pugach and Gibson, 2014). Those fibers essentially suspend the tooth inside the socket, like a bicycle wheel hub is suspended by the wheel spokes. This extra intermediate group of collagen fibers allows extensive tooth movement, like hammock strings would allow for a hammock. This adaptation allows rodents to masticate a wide range of objects without causing dental trauma.
Another structural difference in rodent tooth anatomy is the repartition of dentin and enamel, which differs from that of brachydonts. For a hypselodont tooth to stay sharp, the tooth must be continuously gnawed and worn down, a process called thegosis. However, as mentioned previously, the enamel is an extremely hard tissue that would be laborious to grate. Therefore, in rodents, enamel only covers the surface of the tooth that will not be used for gnawing, i.e. the anterior surface. The diagram above, showing a cross-section of a rodent tooth, illustrates accurately the repartition of dentin and enamel in a rodent tooth. We can identify the pulp in the core of the tooth, which already formed the rootless canal. The pulp is surrounded with dentin and enamel, yet enamel covers only a surface of the teeth. This allows the rodent to wear down the dentin in the back before the enamel in the front. This graded interface of two dental tissues with significantly different hardness levels allows the teeth to wear off in a sharp blade style. As such, the inner surface of the tooth wears off at a specific angle which allows the tooth to stay sharp (“Rat Teeth” | Rat Behavior, 2004).
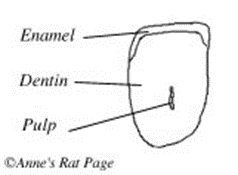
Jaw Movements and Incisor Attrition During Gnawing
During cutting, biting, and pressing, forces are exerted on the cutting edge of the incisor. The sum of these forces represents the cutting force of the incisors. Thus, the teeth must be adapted in shape and in supporting tissues to face these forces.
The upper and lower incisors do not have the same function when cutting and gnawing. The upper incisors are mainly used as an anchor, and move only slightly during incising food, meanwhile the lower incisors perform the gnawing movement (Stefen et al., 2016). The tip of the lower incisor is used as the main cutting tool. The wedge angle of the incisor tip needs to be optimized. For example, it has been shown that for wood cutting, beavers have an optimal wedge angle of 27°. This feature is essential as dentine and enamel are adapted mainly to tolerate compression forces and the wedge angle allows a proper distribution of forces. “The forces acting on the cutting blade of the incisors are transmitted along the location of centers of gravity” (Stefen et al., 2016). Hence, the incisors receive exclusively compressive forces, as the periodontal fibers redirect resulting forces; the compressive stress decreases along the length of the incisor so that the dentin can be reduced.
After cutting the food, the gnawing process continues with the activation of the jaw muscles. During gnawing, the jaw describes movements in which the lower incisors move in a cranial manner “toward the upper incisors and pass behind the upper incisors” (Stefen et al., 2016) as it passes behind the upper incisors, the dentin of the lower incisors is eroded. The diastema is responsible for this arrangement as it maintains the separation between the cheek teeth from the incisors, when the incisors are in occlusion, the cheek teeth are not. This arrangement results from the fact that the distance between the tip of the incisor and the anterior end of the first cheek tooth is longer in the upper jaw than in the lower jaw (Stefen et al., 2016).
The contraction of muscles moving the mandible during mastication and especially gnawing is responsible for the erosion of the inner part of the tooth. Mastication is caused by a lateral shear movement of the jaws in rodents. “The lower jaw cuts back and forth between the peg teeth and the larger maxillary” (Salter, 2007). The function of muscles is to raise the mandible and contract it. Currently, the relative position of each jaw is such that the lower jaw is recessed closed behind the upper jaw. The ever-growing incisors are at the anterior end of each jaw. This resulting structure is the result of an evolutionary mechanism. Muscles activating the jaw developed according to the indefinite growth of the incisors (Cope, 1888).
The growth of the incisors is the cause of a posterior pressure which tends to bring the lower jaw back and to position itself behind the upper jaw. Indeed, the pressure has resulted in pushing the mandible condyle backward in the postglenoid process, resulting in its atrophy and the position of the lower jaw in a posterior position. The absence of the post glenoid process gives freedom to the lower jaw, which can slide back and forth during mastication (Salter, 2007).

The anteroposterior positioning of the jaw then results in the development of muscles whose contraction contributes to incisor attrition (Cope, 1888). The temporal muscle expanded on the ramus allowing a backward upward movement (Cope, 1888). The lower jaw elevation is triggered by the contraction of the deep masseter and anterior temporal during the mastication (Hiiemae, 1971). This movement occurs as soon as the lower incisors meet the superior incisor (Cope, 1888).

The extension of the temporal muscle on the ramus changes its angle of inclination, which causes the internal pterygoid and masseter muscles to stretch in a rather horizontal position causing anteroposterior motion rather than upward (Cope, 1888). Continued activity of internal pterygoid during gnawing is responsible for the “protruded and depressed position of the mandible” (Byrd, 1981).
During mastication, the contraction of three muscle groups allows the mandible to come back to its original position. “The pterygoids and suprahyoid produce depression and forward shift. The suprahyoid and temporal backward shift and elevation of the mandible, while temporal and masseter cause final closure of the mouth starting forward grinding movement” (Salter, 2007). These muscles are particularly involved in producing sharp incisors by rubbing them with a posterior motion (Cope, 1888). Moreover, the more procumbent is the incisors, the sharper the tip becomes as it wears.
Conclusion
Rodents have unique diets, constantly gnawing on materials — a technique that is excellent at wearing down teeth — and have adapted to this challenge over time with ever-growing incisors. The extraordinary rate of growth coupled with a remarkable self-sharpening design highlight the efficiency and ingenuity of their evolution. The versatility of such a design, where what began as a simple chewing device, but evolved to be able to complete such tasks as breaking wood or the thick skin of prey, is clear. Nevertheless, rodents still fall prey to many serious dental conditions that often prove lethal. Despite the risks, the extreme success of the rodent order is proof the ever-growing incisor trait is a massive advantage.
References
Becerra, F., Echeverría, A., Vassallo, A. I., & Casinos, A. (2011). Bite force and jaw biomechanics in the subterranean rodent Talas tuco-tuco (Ctenomys talarum) (Caviomorpha: Octodontoidea). Canadian Journal of Zoology, 89(4), 334-342. doi:10.1139/z11-007
Behavior, R. (2004). Rat Teeth. Retrieved from http://www.ratbehavior.org/Teeth.htm
Byrd, K. E. (1981). Mandibular movement and muscle activity during mastication in the guinea pig (Cavia porcellus). Journal of Morphology, 170(2), 147-169. doi:https://doi.org/10.1002/jmor.1051700203
Capello, V. (2008). Diagnosis and Treatment of Dental Disease in Pet Rodents. Dentistry, 17(2), 114-123. doi:10.1053/j.jepm.2008.03.010
Cook, M. J. (1965). The Anatomy of the Laboratory Mouse: Academic Press. Retrieved from https://books.google.ca/books?id=c7hqAAAAMAAJ
Cope, E. D. (1888). The Mechanical Causes of the Origin of the Dentition of the Rodentia. The American Naturalist, 22(253), 3-13. doi:10.1086/274627
Cox, P. G., Rayfield, E. J., Fagan, M. J., Herrel, A., Pataky, T. C., & Jeffery, N. (2012). Functional Evolution of the Feeding System in Rodents. PloS One, 7(4), e36299. doi:10.1371/journal.pone.0036299
Dictionary, M.-W. (Ed.) (n.d.). shear. In. merriam-webster.com: Merriam-Webster. Retrieved from https://www.merriam-webster.com/dictionary/shear
Druzinsky, R. (2015). The oral apparatus of rodents: Variations on the theme of a gnawing machine. In (pp. 323-349).
Freeman, P. W., & Lemen, C. A. (2008). A simple morphological predictor of bite force in rodents. Journal of Zoology, 275(4), 418-422. doi:https://doi.org/10.1111/j.1469-7998.2008.00459.x
Froberg-Fejko, K. (2014). Give a rat a bone: satisfying rodents' need to gnaw. Lab Animal, 43(10), 378-379. doi:10.1038/laban.611
HIIEMAE, K. (2008). The structure and function of the jaw muscles in the rat (Rattus norvegicus L.): III. The mechanics of the muscles. Zoological Journal of the Linnean Society, 50(1), 111-132. doi:10.1111/j.1096-3642.1971.tb00754.x
Maestri, R., Patterson, B. D., Fornel, R., Monteiro, L. R., & de Freitas, T. R. O. (2016). Diet, bite force and skull morphology in the generalist rodent morphotype. Journal of Evolutionary Biology, 29(11), 2191-2204. doi:https://doi.org/10.1111/jeb.12937
Myers, P., Espinosa, R., Parr, C. S., Jones, T., Hammond, G. S., & Dewey, T. A. (2020, 2021). Structure and placement of individual teeth. Retrieved from https://animaldiversity.org/collections/mammal_anatomy/tooth_structure/
Osofsky, A., & Verstraete, F. (2006). Dentistry in Pet Rodents. Compendium on Continuing Education for the Practicing Veterinarian, 28.
Pocketdentistry. (2015). Enamel: Composition, Formation, and Structure. Retrieved from https://pocketdentistry.com/7-enamel-composition-formation-and-structure/
Pugach, M. K., & Gibson, C. W. (2014). Analysis of enamel development using murine model systems: approaches and limitations. Frontiers in Physiology, 5(313). doi:10.3389/fphys.2014.00313
Reznikov, N. (2020). Properties of Biological Matter: Mechanical Properties of Hard Material. In. Montreal: McGill University.
Rinaldi, C., & Cole, T. M. (2004). Environmental seasonality and incremental growth rates of beaver (Castor canadensis) incisors: implications for palaeobiology. Incremental Growth in Vertebrate Skeletal Tissues: Paleobiological and Paleoenvironmental Implications, 206(3), 289-301. doi:10.1016/j.palaeo.2004.01.008
Salter, R. (2007). Rabbit and Rodent Dentistry. In. Glen Waverley, Australia. Retrieved from https://www.vin.com/apputil/content/defaultadv1.aspx?id=3860700&pid=11242&
Srot, V., Bussmann, B., Deuschle, J., Pokorny, B., Watanabe, M., & van Aken, P. A. (2017). Magnesium-Supported Continuous Growth of Rodents' Incisors. Microscopy and Microanalysis, 23(S1), 1320-1321. doi:10.1017/S1431927617007267
Stefen, C., Habersetzer, J., & Witzel, U. (2016). Biomechanical aspects of incisor action of beavers ( Castor fiber L.). Journal of Mammalogy, 97(2), 619-630. doi:10.1093/jmammal/gyv209