A Ton of Mechanical Advantages: Analyzing the Remarkable Structure of Elephant Limbs
Andrea Khoury, Nikki Lal, Spencer Levine, Laura Camila Penuela Cardenas
Abstract
Elephants are the largest land animals in the world. With adults weighing between 6 000 and 13 000 kilograms, one elephant weighs just as much as an empty semi-trailer! In fact, calves already pack a whopping 200 lbs right from the start of their life. Carrying such an enormous size and weight undoubtedly leads to some important evolutionary adaptations. The purpose of our research is to delve into the structure of elephant limbs — the beams that support this majestic beast through all its journeys. From the perspective of materials and mechanics, the paper discusses important, unique features that allow these limbs to perform their function, including foot cushions, pre-digits, and vibration sensitivity. Further, the research moves into defining characteristics that distinguish fore limbs from hind ones, such as their bone structure and mechanics, and the limbs of both main species of elephants, the African (referred to as AFE in the paper), Loxodonta africana, and the Asian (ASE), Elephas maximus. Finally, the paper introduces an exciting concept of how, mechanically speaking, the trunk acts like a limb and can be analyzed as such.
Introduction
Circa 400 million years ago, the first fish began leaving their water habitat and evolving a mechanism to survive on land. Among crucial respiratory system changes that needed to be made, another important shift took place: the development of limbs from fins. All descendants of these ancient “land fish” are known as tetrapods, which means “four legs”. All tetrapods are vertebrates and, interestingly enough, an organism does not even need to have or use four limbs in order to be a member of the Tetrapoda superclass. Over hundreds of millions of years, these limbs have continued to evolve and adapt to each species' environmental challenges, but still retain many of the same elements. This type of evolution is called divergent or homologous.
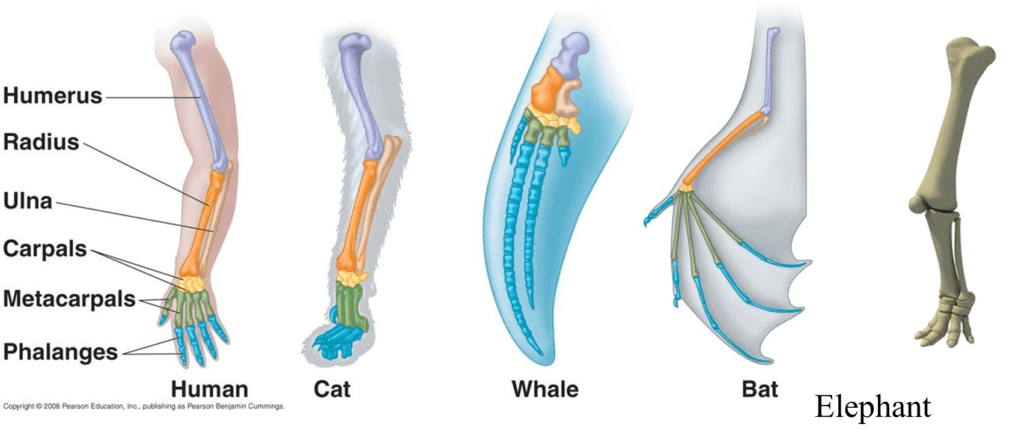
These bone structures retain some very important characteristics, such as a single larger bone at the top of the limb which separates into two smaller structures at a joint and finally splits into 5 digits at a second joint. This prompts the question: how can this model, which is used in bats to fly, also used in elephants, the largest land animal on Earth?
In this paper, we will focus on the incredible mechanical adaptations that enable elephant limbs to provide support for the massive body they carry. Evidently, limbs are made up of much more than bones. Muscles, tendons, ligaments and cartilage play incredible roles in providing mechanical strength, durability and specific advantages to the limbs where they are found. Furthermore, it would be incomplete to discuss these limbs without considering how they differ within elephants. These differences can be found between fore and hind legs and between AFE and ASE species. Finally, the research will divert from the discussion of typical limbs onto the intriguing topic of elephant trunks as limb-like structures and their potential use in the engineering field.
Biological Characteristics of Elephant Limbs
A Soft Cushion
Supporting the weight of the largest terrestrial animal sounds at first challenging for the elephant's limbs but this role is mostly carried by large subcutaneous cushions which are important in the process of distributing forces during weight bearing, but also for storing or absorbing mechanical forces (Weissengruber et al., “The structure of the cushions in the feet of African elephants”). In addition to their mechanical properties, the elephant's foot cushions are very sensitive structures.
The reason why elephants walk quietly is due to the fibro elastic layer providing a spongy cushion on the bottom of the foot. This allows the foot to mold over objects on the ground, thereby smothering the objects beneath it (Weissengruber et al., “The structure of the cushions in the feet of African elephants”). This feature, however, is not the primary purpose of the elephant's foot cushions.
These cushions in the elephant's feet were at first examined through Computed Tomography (CT) and Magnetic Resonance Imaging (MRI) which are two means of standard anatomical and histological techniques. They consist of white and yellowish strands of fibrous connective tissue forming larger metacarpal/metatarsal — any bones of the hand and foot — and digital compartments and smaller cavities which were filled with body fat, also known as adipose tissue.
The compartments are located between structures of locomotor apparatus covering the bones parlmarly/plantarly and the thick sole skin (Weissengruber et al., “The structure of the cushions in the feet of African elephants”) (Fig. 2). Additionally, vascular supply and numerous nerves course down into adipose tissues and are found throughout the cushions. These vessels form a network which is visible proximal to the skin of the sole (Weissengruber et al., “The structure of the cushions in the feet of African elephants”).
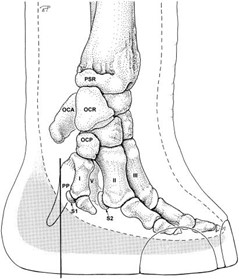
Furthermore, the cushions are formed by several proteins such as collagen and reticulin but also by elastic fibers which give elasticity to tissues in the body allowing the balls of fat to spread out as much as 20 %. When loaded under compressive forces, the cushion expands medially, laterally and palmarly/plantarly (Weissengruber et al., “The structure of the cushions in the feet of African elephants”). This expansion on each limb greatly lightens the weight felt by the individual's large mass.
When looking at microscopic findings (Fig. 3), we denote that the sole of the elephant foot is covered by a thick keratinized squamous epithelium, the epidermis, which lies on a massive layer of dense connective tissue forming the dermis. The dermis represents a thick, organized layer of interwoven and very tightly packed bundles of collagen fibers with only few interspersed elastic fibers. The dermis is very rich in blood vessels and nerves. Numerous large arteries and veins lie within the connective tissue (Weissengruber et al., “The structure of the cushions in the feet of African elephants”).
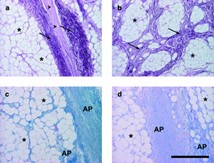
Pre-digits
Elephants usually roam over 18 000 miles per year. To perform these extremely long trips, they can count on their atypical suspension systems: they walk on the tips of their toes but have heels padded with fat which help them support their weight. They also have expanded structures in the fat pads of their fore- and hindfeet (O'Connell et al., 1999).
In fact, their five conventional toes point forwards, giving elephants a tip-toed stance, whereas there is a sixth toe facing backwards which serves as a stabilizer during long walks (Fig. 4).
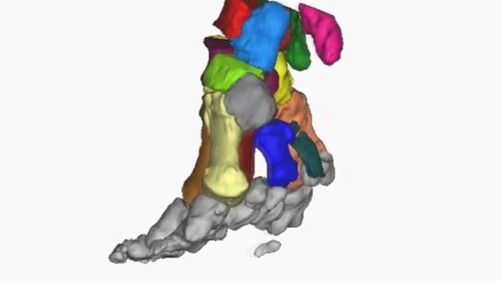
The mystery of this sixth toe goes back to 1706 when the first elephant was dissected by a Scottish surgeon. At that time, scientists did not understand the utility of this pre-digit. It became clearer when they encountered several fossils (Hutchinson et al., “From Flat Foot to Fat Foot”). Analyses of the fossils determined that this “pre-digit” evolved about 40 million years ago, at a point when early elephants became larger and more land based. When the first elephants appeared 55 million years ago, they had relatively flat paws and their ankles rested on the ground while the pachyderms — large mammals such as elephants, rhinoceros and hippopotamus — became bigger.
This additional toe is in fact a piece of cartilage that grows and gets hardens over time, leading to the creation of a bone which acts as a crutch inside the foot. This extra digit is between 5 and 10 centimeters long (Fig. 5). Moreover, the ones on the hindfeet seem to have joints (Hutchinson et al., “From Flat Foot to Fat Foot”).
By loading the feet with weights inside a CT scanner, researchers showed that the sixth toe acts as a strut that stiffens the back of the fat pad. It helps the pad to support the elephant's weight without collapsing down too far. This also explains why the toe gets stronger as the animal ages and gets heavier (Hutchinson et al., “From Flat Foot to Fat Foot”).
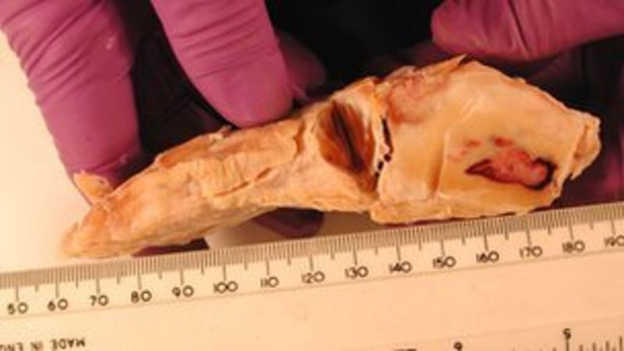
This sixth toe is a great example of the repurposing of a structure by evolution to have more suitable characteristics. It comes from one of the massive, small bones found near the tendon thickness — also called sesamoids — which is turned into a functional finger instead of evolving a new one. In other words, elephants co-opted sesamoid bones into a role as false digits and used them for support as they changed their foot posture.
Seismic Sensitivity
Elephants are huge and impressive animals. Their most noticeable physical aspects would be their two enormous and wide ears. However, these massive mammals have two distinct ways of communicating: by ordinary soundwaves rippling through the air, and by vibrations transmitted through the ground to another elephant's sensitive toes.
The human's hearing range goes from 20 Hz to 20 KHz. Sounds bellow that range are called infrasonic. Fascinatingly, that infrasonic range is where most of elephant's communication occurs (between around 14 and 35 Hz) (O'Connell et al., 1999). Through each long trumpeting call, high and low frequency waves are spread. The high frequency sounds reach animals within a close radius — a mile or so, — whereas low frequency sounds generate seismic waves in the ground and reach recipients that are far from them (at least 5 or 6 miles but it can reach up to 12/13 miles away) (“Seismic communication” | ElephantVoices, n.d.).
Infrasound allows elephants to communicate over impressively long distances. Lower frequency sounds have higher wavelengths (widely spaced ripples instead of closely spaced ripples associated with high frequency sounds) (Fig. 6). Since they vibrate more slowly, they retain energy longer too, meaning they can travel farther before fading out. Moreover, low-frequency sound waves are larger than most barriers in their paths avoiding being blocked or absorbed by obstacles (“Seismic communication” | ElephantVoices, n.d.).
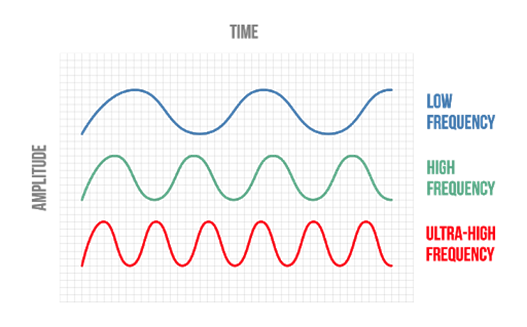
Through these ground vibrations, elephants may raise long-distance alarms, offer advice and even advertise love yearnings (“Acoustic Communication” | ElephantVoices, n.d.).
The recipients receive the information through their feet and toes, behind which lie pads of acoustically sensitive fat. As a matter of fact, when elephants sense the low vibrations, they press their heavily padded feet to the ground which spreads out their huge balls of fat, also called digital cushions, as much as 20 % (Weissengruber et al., “The structure of the cushions in the feet of African elephants”). There are dozens of touch receptors in the elephant's foot called Pacinian corpuscles. The receptors intercept these vibrations and send a signal to the brain. Then, microseconds after, the brain identifies the information and processes it in the auditory cortex area, like regular sounds. The feeling becomes hearing. The vibrations are spread through the elephant's skeleton all the way to the ear (Weissengruber et al., “The structure of the cushions in the feet of African elephants”).
Several studies demonstrated that the same danger call can be perceived differently when communicated via low or high frequency waves. If the message is from high frequency sounds, this means that it is spread through the air, hence, the elephants would need to flee a nearby threat (“Acoustic Communication” | ElephantVoices, n.d.). In that case, these smart animals would act quickly and would seem panicked. Whereas, if it is from low frequency waves, the information comes from the ground, meaning that the danger is a long distance away. This allows the elephants to react slowly and calmly to the danger. In these experiments, the low frequency sounds were transmitted and spread with an instrument called a shaker (“Studying Elephant Communication” | biointeractive | YouTube, 2017). This device is usually used to play back the low frequency from movies. Other studies demonstrated the utility of long-distance calls by tracking the movements of herds with radio collars. The outputs of the research indicate that family groups were able to spend days coordinating their movements, even when they were separated by as much as 3.1 miles! (“Studying Elephant Communication” | biointeractive | YouTube, 2017)
Furthermore, the elephant's footsteps transmit useful information about its size and whereabouts to other elephants (“Seismic communication” | ElephantVoices, n.d.) (Fig. 7).
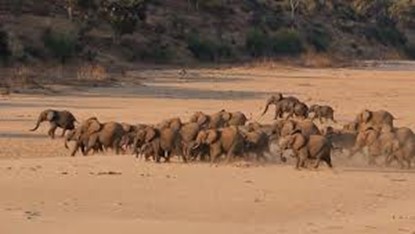
Other experiments were conducted, linking weather variations to changes in the herds' attitude. As a matter of fact, rainstorms are very noisy especially at low frequencies, allowing elephants to forecast the weather. In fact, weeks before dry season turns to the rainy season, elephants tend to switch from short-distance foraging to long-distance migration or vice-versa. The reasons for these behaviors are still unclear, but we can easily articulate that the elephants can hear storms coming (“Seismic communication” | ElephantVoices, n.d.). Incredibly, elephants' ability to feel seismic signals was first brought to public attention when trained elephants in Thailand moved to higher ground just before a tsunami struck, saving themselves and their owners (Jackson, 1918, as cited in O'Connell-Rodwell, 2007).
Seismic signals may also play an important role in the elephant's reproduction as they send seismic vibrations to potential mates. This allows them to better reproduce and keep this innovation.
The elephant's communication is still unclear to humans. However, by understanding its functions and mechanics, humans are getting closer to using these properties to help babies born with hearing impairments, but very sensitive to vibrations for the first 11 months (“Seismic communication” | ElephantVoices, n.d.).
Comparison between Fore and Hind Limbs
Many of the elephant's incredible abilities are accorded to the peculiar design principles of its limbs. Hence, it is primordial to delve into the architecture and function of the fore and hind legs of the largest living terrestrial animal to have a better understanding of it.
Elephants' legs are almost ‘columnar'. As a matter of fact, they have very little angulation since “the articular surfaces are in line with the axis of the leg”. This allows the fore and hind members to avoid unnecessary exertion during locomotion (Ramsay and Henry, 2001). In addition, elephants' leg bones have a high density (Tefera, 2012) and are made up of cortices which enclose cancellous bone lacking marrow cavity. The tibia and femur do not lack medullary cavity, however. It is the combination of bone placement and structure that make the limb bones stable and capable of resisting more pressure, thus permitting elephants to not only bear heavy weights, but also to remain upright for long periods of time and sleep whilst they are standing (“Asian elephant” | Smithsonian's National Zoo and Conservation Biology Institute, n.d.).
Regarding weight distribution, around 60 % of the elephant's weight is supported by the forelimbs whereas approximately 40 % is supported by the hind legs. This was proven by a study carried out in 2010 on the “Biomechanics of locomotion in Asian elephants”. In the latter, it was found, through observations of the movement of the center of mass (COM) of 34 Elephas Maximus over the speed range of these mammals using force platforms, that the fore legs “support almost 2/3 of the body weight both dynamically and statically” (Genin et al., 2010).
Moreover, the structure of elephants' feet must be discussed as it contributes greatly to the identification of these mammals besides letting them fulfill various functions. Starting off by taking a close look at the sole, it is noticeable that the fore feet are round-like whilst the hind feet are smaller and oval-shaped; the larger size of the fore sole is theorized to be a result of the resting of most of the body weight on the fore legs (Tefera, 2012). What is more, the sole is “rigged and pitted which gives the elephant stability when walking over a variety of terrains” (“Elephant Anatomy” | Animal Corner, n.d.). This characteristic becomes extremely useful for these mammals whenever they climb up and down steep slopes or walk on slippery surfaces, for example.
Furthermore, the dimension of the footprint provides a great amount of information concerning the age, sex and height of an elephant. In fact, the measured length of hind prints gives a quick and quite accurate age estimate where the print was clear according to a 1992 study in a group of elephants in Kenya's Amboseli National Park (Table 1).
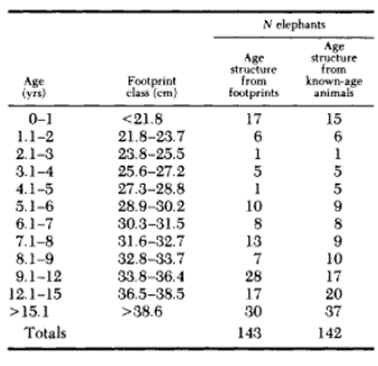
A less accurate method that roughly determines an elephant's age is how defined the print is on the soil as “younger elephants leave a more defined footprint than older elephants that might have smoother ridges and well-worn heels” (“African Elephant” | African Wildlife Detective, n.d.). In addition, it appears that males leave a rather ovoid mark whereas females' is more circular (“Elephant Anatomy” | Animal Corner, n.d.). Moreover, it was remarked on a 2008 study on the Asian elephants of Cat Tien National Park in Vietnam that an elephant's shoulder height corresponds to approximately twice the circumference of its footprint (Table 2). The print that elephants leave behind creates a small pond in which microorganisms and certain invertebrate species reside, therefore creating an essential habitat for the latter (Remmers et al., 2016).
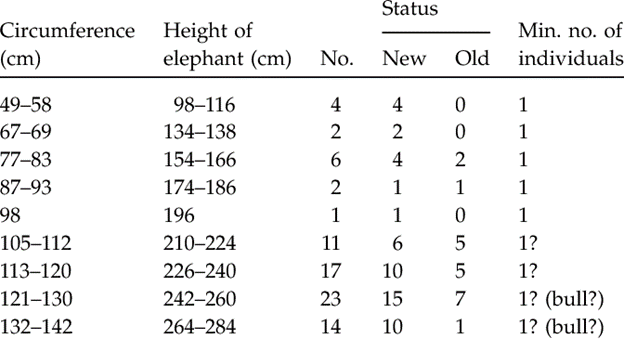
Additionally, the design of elephants' feet makes the soles expand under the mammals' weight and contract when the weight is removed. This allows for the animal's mass to be distributed in a uniform manner and explains why these large mammals can easily move whilst walking in deep mud. Indeed, suction is reduced as the elephant removes its foot from the mud since the foot's circumference becomes smaller (“Elephant Anatomy” | Animal Corner, n.d.). Elephants are considered as digitigrade for they walk on their digits. It is the way in which their feet are structured that allows them to walk as such. Fig. 8 corresponds to an analysis effectuated in 2008 which illustrates the movements of limb segments and joints of an African elephant (Ren et al., “The movements of limb segments”).
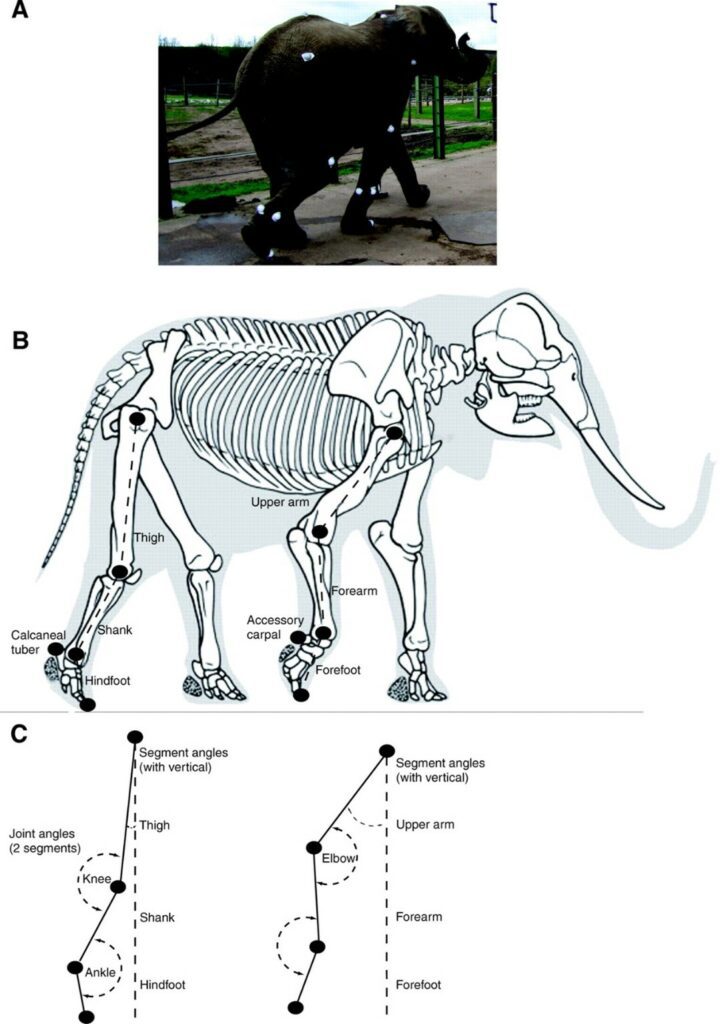
The following subsections will explore details of the fore and hind feet.
Fore Foot
Also referred to as the manus, the fore foot is comprised of 5 digits (I-V), 8 carpals (accessory carpal, four carpals (C1-4), intermediate carpal, radial carpal and ulnar carpal) 5 metacarpals and a prepollex (Miller et al., 2007).
Carpals: the eight carpal bones are arranged in two rows: proximal and distal. The proximal row is comprised of the accessory, intermediate, radial and ulnar carpals whilst the distal row is made of the four carpals (C1-4).
Metacarpals: the metacarpals are positioned almost vertically (Miller et al., 2007).
Digits: among the five digits, digit III is the longest and V is located below the ulna. Digits II, III, and IV each have three phalanges whereas digit I has a single phalanx (Ramsay and Henry, 2001). “The more horizontal orientation of the manual phalanges also suggests that they incur relatively greater bending loads than in the pes” (Weissengruber et al., “The structure of the cushions in the feet of African elephants”)
Prepollex: according to a research conducted in 2007 on how the musculoskeletal anatomy of the fore and hind feet of elephants changes with the latter's development, the prepollex as well as the prehallux (structure of pes) “remain relatively robust in comparison with other mineralized tissues of the feet, strongly supporting the hypothesis that they play a major role in foot support” (Miller et al., 2007).
Hind Foot
Equally referred to as the pes, the hind foot is comprised of 5 digits (I-V), 4 tarsals (T1-4), a central tarsal, 5 metatarsals, a talus, a calcaneus, a prehallux, and paired sesamoids (Miller et al., 2007).
Digits: all digits radiate in a craniocaudal orientation. III and IV have three phalanges whereas V has only two. The length of the third digit is the same as the length of digit III of the manus. For the pes, digit V is located below the fibula (Ramsay and Henry, 2001). Moreover, digits “II, III and IV act like the three supports of a tripod”. This special orientation of the latter mentioned digits implies that they must have a higher resistance to bending and torsion, and be more robust cross-sectionally (Miller et al., 2007).
Tarsals (T1-4 + Central): the distal row of tarsals is comprised of T1-4. The latter have the shape of a wedge. The central tarsal is the only bone of the second row of tarsals (Ramsay and Henry, 2001).
Metatarsals: there are five metatarsals in the pes and they each have a different orientation with respect to the vertical as digits “I and V are the most vertical and digit III the most horizontal” (Miller et al., 2007). In addition, the arch-like arrangement of the metatarsals makes them useful for shock absorption (Weissengruber and Forstenpointner, 2004).
Tarsus: the tarsus is made up of seven bones that are laid out in three rows. It has a disc-like shape and, along with the calcaneus, it comprises the proximal row (Ramsay and Henry, 2001).
Prehallux: this is a segment made of cartilage that extends in a distal manner from the first metatarsal and T1. It also joins the sole medial to the midline (Ramsay and Henry, 2001).
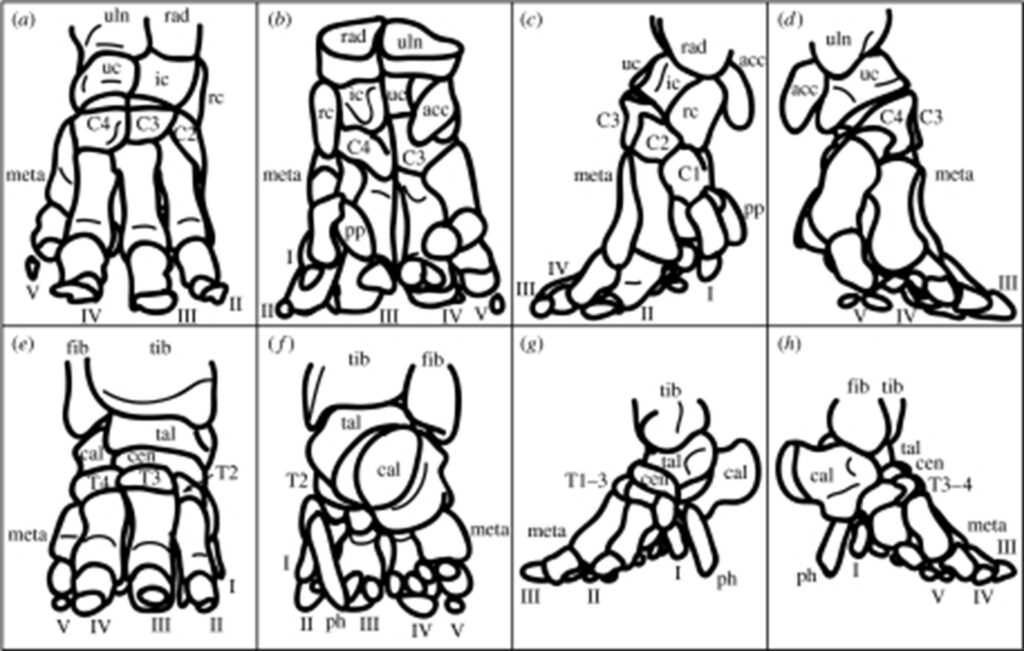
A fascinating remark that was made through the ontogenic analysis of 2001, is that most bones of the fore and hind feet as well as the tendons of the manus either conserve their shape or become slenderer as the elephant develops. Nevertheless, the tendons of the pes become notably more robust with time, which potentially indicates a functional adaptation to heavy-load bearing (Miller et al., 2007).
On another note, in a study carried out in 2004 by G.E. Weissengruber and G. Forstenpointner from the University of Veterinary Medicine Vienna, the hind members of two female and four male African elephants were dissected to further investigate the arrangement of the muscles of the crus and pes of the Loxodonta africana. This study revealed certain indispensable muscular characteristics of these mammals. For instance, it is presumed that “the oblique position of the tendons of the Mm. tibialis caudalis and fibularis brevis […] helps to maintain tension in the arch of the foot”. Furthermore, well-developed muscles such as the M. soleus were found to be required for specific movements of the limbs during locomotion. Additionally, the “tendon-like facial sheet” that covers the M. soleus appears to make it contribute to the support of “the articulatio tarsi in the resting position as part of the stay apparatus”. What is more, there is a tendon of the M. semimembranous that fuses with the articulatio tarsi which is theorized to contribute to foot lifting (Weissengruber and Forstenpointner, 2004).
Other research have focused on examining different parts of the limbs of an elephant such as the knee. Concerning the latter, its “highly congruent articular surfaces and only small menisci which are less vulnerable to traumatic forces” aid in the support of the elephants' weight when the knee is completely extended (Weissengruber et al., “The elephant knee joint”).
To better evaluate the mechanics of movement of elephants, the focus will now be shifted towards their walking pattern and the power in their limbs.
As observed by Hutchinson et al., the fore legs of an elephant have a movement characteristic of walking at high speeds (15 – 25 km/h) whilst its hind limbs have a movement characteristic of running. In addition, it was noticed that the mammals always maintain one foot in contact with the ground regardless of how fast they are going (Hutchinson et al., “Are fast-moving elephants really running?”). Another captivating remark related to elephants' footfall pattern is the use of a lateral sequence. This means that when there is limb motion, the hind leg of one side is followed by the fore limb of the same side (Genin et al., 2010).
Ren et al. concluded, at the end of their study on how elephant limbs support the mammal's weight and provide them with the necessary thrust to cause locomotion, that the fore legs do twice as much work as the hind members; all four limbs are equally used for braking and propulsion, nonetheless. Additionally, “the forelimbs' power contributions closely correspond to the total mechanical power, whereas the hindlimbs' power contributions often are out of phase with total power”. Hence, the locomotor mechanics of elephants are primarily controlled by their fore legs (Ren et al., “Integration of biomechanical compliance”).
Elephant Limbs across Species
Today on Earth, there are two main species of elephants: the African elephant (AFE), Loxodonta africana, and the Asian elephant (ASE), Elephas maximus (de Silva and Wittemyer, 2012). AFE live in desert and forest-like environments, particularly the savanna or rainforests. ASE occupy Asian countries like India, Sri Lanka, Thailand, and more (“Elephant” | Science Encyclopedia, n.d.). They are accustomed to forest environments and, sometimes, domestic life.
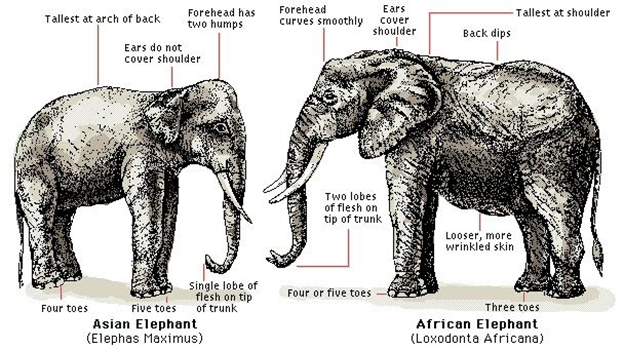
The AFE and ASE species diverged about six million years ago, with similar ancestors being mammoth species (de Silva and Wittemyer, 2012). As mentioned, the habitats of these elephant species differ very much, for example in weather patterns, average rainfall, and available resources. Nevertheless, the elephant species have similar niches in their distinctive habitats because they are among the largest herbivores (de Silva and Wittemyer, 2012). Generally speaking, the elephant species do exhibit many similarities and differences in their physical appearance and social networking. AFE are larger than ASE, with bigger ears and longer tusks (“What's the Difference between Asian and African Elephants? And 8 Other Elephant Facts” | WWF, n.d.). In terms of their social organization, both species are matriarchal, and their distinct groups are made primarily of females and their offspring; the male elephants tend to live more individually than females. African elephants, however, interconnect with large numbers of other AFE groups, forming a very great network of elephant groups (de Silva and Wittemyer, 2012). ASE do not connect as much with other groups of their species and remain social in smaller groups of mother-calf relationships (de Silva and Wittemyer, 2012).
These social factors, among other habitat factors, affect how much these elephants use their limbs; for example, Sri Lankan elephants historically have very few predators aside from humans. Because of this, they tend to stay in the open air more than other elephant groups and do not need to reach high speeds for self-protection (de Silva and Wittemyer, 2012). Contrarily, AFE must worry about lions and other predators striking their calves. They are, therefore, more in need of high energy outputs in the limbs to protect their families (de Silva and Wittemyer, 2012).
For a pair of species that live in drastically different environments, the African and Asian elephants still use their limbs in the same capacities and with similar kinetic patterns. The knee joint, a crucial joint for the movement of elephants, is very similar in both species (Weissengruber et al., “The elephant knee joint”). Alternatively, the possible extension, muscle structure, and patterns of locomotion of the semi-plantigrade elephants are more like the plantigrade humans than larger herbivores, despite having different locomotive systems. Because of the limited flexion of the elephants' knees and their column-like legs, elephants are quite different from similarly sized animals, like hippopotamuses and rhinoceroses (Weissengruber et al., “The elephant knee joint”).
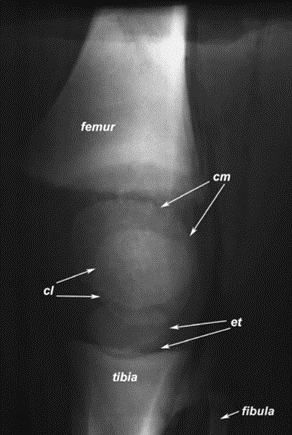
Between African and Asian elephant species, the locomotive kinematics are comparable as well. Maximal speed is the fastest speed an elephant reaches in their lifetime, typically unfrequently attained (Hutchinson et al., “The locomotor kinematics of Asian and African elephants”). A trend concluded in Hutchinson et al.'s research is that elephants must exert themselves incredibly to reach any relative fast speed and, therefore, one cannot calculate or assume what the elephant's maximal speed really is. The study instead measured what the authors call “near-maximal speed” to refer to any fast speeds (over 5 or 6 m/s) the elephants attain. African and Asian elephants both consistently express the same footfall pattern, which remarks how the limbs move while locomoting. The highest near-maximal speed was seen in Thai elephants and is around 6.8 m/s. Most other elephants measured a near-maximal speed of around 6 m/s as well, and the researchers concluded that < 7 m/s is a likely near maximal speed of all African and Asian elephants (Hutchinson et al., “The locomotor kinematics of Asian and African elephants”). Although there were slight disparities between some factors of the data collection in this study, the overall comparative conclusion of the species is that there are minimal biological differences in the kinematics of these two species.
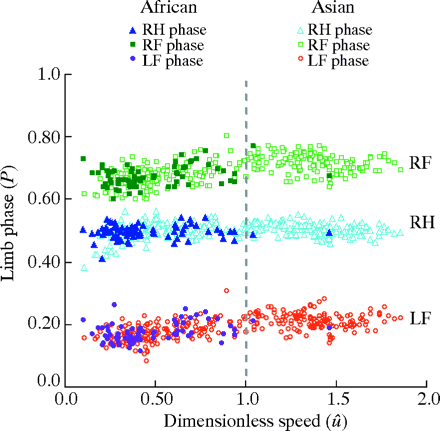
African elephants and Asian elephants are represented by the symbols in the legend. RF = right front feet, RH = right hind feet, LF = left front feet. This plot demonstrates how similar the limb phases are between the different species.
The 5th limb?
Based on the evolution and bone structure models discussed before, it can be stated that elephants certainly have four limbs. However, intuitively, when analyzing these animals, one can see many similarities between the functionality of human arms and that of elephant trunks.
To begin this inspection, we need to understand exactly what the trunk's purpose is: a nose. With over 40 000 muscles and connecting to sensors encoded by over 2 000 olfactory receptor genes, these noses are one of the most sensitive and flexible in the world (“What's Inside An Elephant Trunk?” | Science Insider | YouTube, 2019) (Fig. 13).
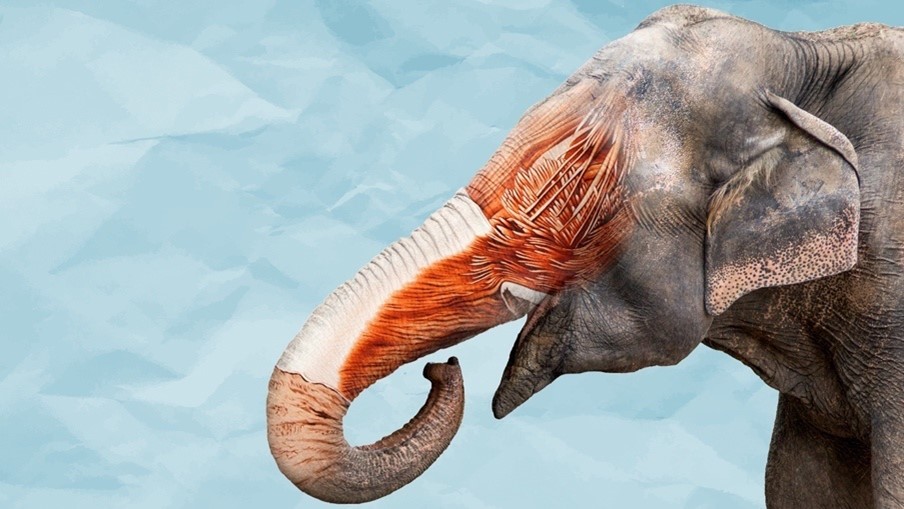
For comparison, humans have around 650 bones in our entire body. These elongated snouts are referred to as muscular hydrostats because they are entirely made of muscle and can manipulate food and other items without any skeletal support (“Muscular hydrostat” | Wikipedia, n.d.). There are no bones nor joints in the structure, allowing it to be incredibly flexible and act similarly to a human arm.
While you may never find a wild elephant painting a self-portrait in the wild, you will find an elephant using its snout to eat, drink, wash itself, and breathe while swimming underwater (like a snorkel).
With such incredible range of motion and copious muscle fibers, an elephant's trunk can lift hundreds of pounds with ease while also having the ability to control its force exertion so precisely so as to not crack a tortilla chip (“What's Inside An Elephant Trunk?” | Science Insider | YouTube, 2019). In one study, researchers determined that elephants use their abundance of muscles to form joints in their trunks and compress/solidify their food so that it can be easily picked up and eaten (Wu et al., 2018). This same study determined that elephants control precisely how much compressive force is exerted depending on what is necessary. This intuitive mechanism saves energy and maximizes mechanical efficiency. As food pieces get smaller, they become harder to compress and hold together. This leads to the first order inverse proportionality seen in Fig. 14 between applied force by trunks and food size.
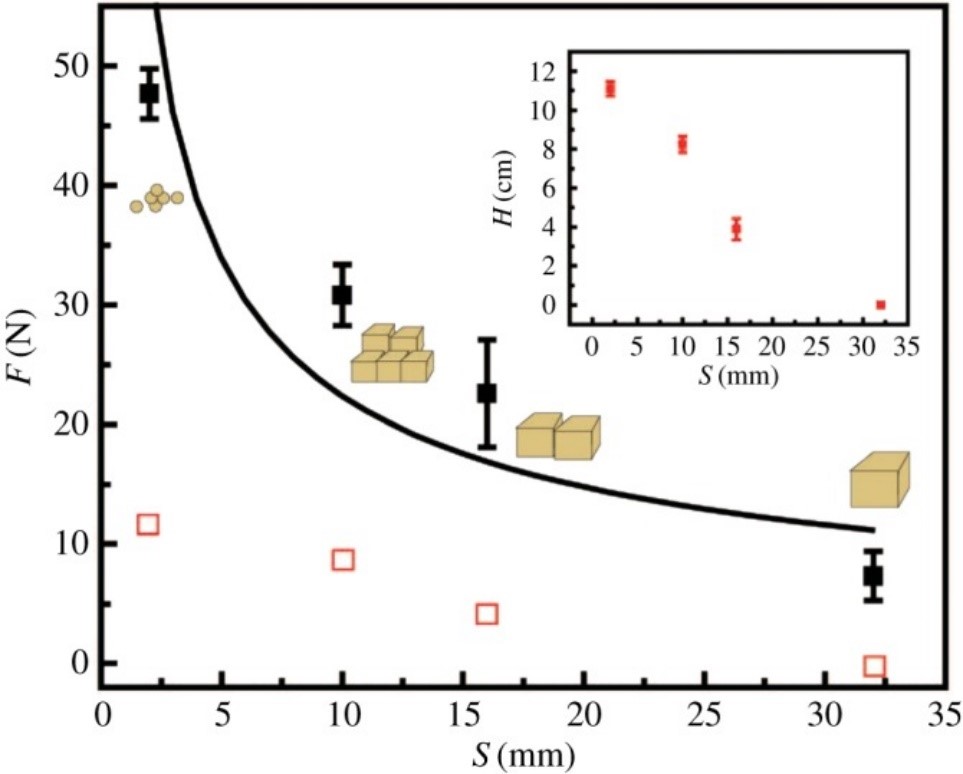
All this information is pertinent because it demonstrates that trunks are not simply noses, snorkels or kitchen utensils; rather, they are an honorary 5th limb. One possible counterargument would be to say that limbs need to support the weight of the creature. However, this logic is flawed, because human arms are certainly considered limbs even if they are not used in the traditional way that other animals show.These muscular structures truly demonstrate — and surpass — abilities that are seen in human arms and should at the very least be considered as functional limbs.
Due to its incredible versatility as a functional limb, elephant trunks are a source of great intrigue to engineers. Evidently, perfectly recreating such a densely packed organ is extremely difficult and time consuming. As a result, engineers have taken a couple of interesting approaches to biomimicry — using nature's versatile masterpiece to solve human problems. In this case, the potential lies in robotic/prosthetic arms that are not limited in the directions that they can move while also being capable of carrying large loads. One remarkably interesting model of a robot elephant trunk involves using springs and cables to mimic the dense packing of muscles and having many small segments each with 6 degrees of freedom (d.o.f.) for a total of 48 d.o.f. (Fig. 15).
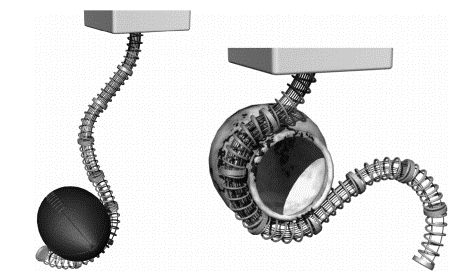
The springs provide elastic flexibility and compression loading strength while the cables provide mechanical strength to the structure. Like a real elephant, when the springs are unloaded and stretched, they can provide a delicate force to not damage fragile objects. With proper force calculations and coordination of the motion of the prototype by a computer, the application of this design could revolutionize robotics/prosthetics industries.
Conclusion
Throughout the paper, we have explored different peculiarities linked to the elephant's limbs but more particularly, we discovered the main roles they play in supporting the weight of this large mammal. The cushions, yellowish strands of fibers, act as softeners and are major contributors in the distribution of the forces thanks to their ability to expand and let the information travel through a multitude of vessels. Moreover, the recent discovery of “sixth toes” in the elephant's feet explained reasonably the stability elephants have when performing long walks. As a matter of fact, these pieces of cartilage, which evolved into bones, are essential in the limbs' mechanics. Furthermore, discoveries lead to understanding better the elephant's communication skills. In fact, through low frequency sounds emitted from the trunk and spread through the ground, these mammals can communicate with each other over several miles. The recipients receive the information in their feet, then, the cushions intercept and transmit it to the brain which processes it in the auditory cortex. Elephants are also able to forecast the arrivals of rainstorms and earthquakes because of the same properties.
What is more, we focused on the design principles of the mammal's limbs to be able to better comprehend how specific limb-related functions can be carried out. To do so, we first examined the general architecture of the legs, which showed that most of the weight is supported by the forelimbs and gave us some insight into how elephants can, amongst others, stand for a long time without expending too much energy. Then, we began looking more closely into the structure and design of the feet by studying the dimensions of the soles as well as the specific arrangement of bones, tendons, and muscles in both the manus and pes. The shape and size of the footprints proved to contribute not only to the identification of elephants, but also to the creation of habitats for other organisms. The ‘layout' of the bones and the musculature of the fore and hind feet demonstrated its importance for shock absorption, foot support, maintaining the tension in the arch foot, heavy-load bearing, and foot lifting. Later, we investigated the architecture of the knee and saw how it was substantial to the bearing of heavy weight. Afterwards, we explored the walking pattern and the power in the limbs, which revealed that elephants mostly rely on their fore legs for locomotion.
Further, we got to compare African and Asian elephants. Although there are environmental differences in how the elephants interact with each other and with predators, the elephants use their limbs for the same reasons and mechanically in the same capacities. With near-maximal speeds of around 6 m/s, African and Asian elephants can run quite fast from a young age, but do not exert themselves to near-maximal speeds unless necessary.
Finally, we looked at the concept of elephant trunks as a 5th limb and how this structure can be applied in engineering fields. We saw how the incredible range of motion and constitution of this structure resembles — and exceeds — some capabilities of traditional limbs. While this structure is difficult to replicate mechanically due to its natural composition of over 40,000 muscles, engineers have made many strides in understanding and mimicking this complex organ. As we gain more insight as to how the trunk is formed and how it functions, we will continue to get a more and more vivid image of how this marvelous structure works and how it can be used to help solve engineering problems.
If there is one main takeaway from the topic of elephant limbs, it would be that nature has a beautiful way of making structures have many different functions. Whether it be foot cushions that allow communication or a nose that can give you a shower, millions of years of evolution have resulted in an extremely efficient system that enables such a massive land animal to thrive. While this paper covers a lot of information, there is always room for future research. Although there may be a lot of details we understand, we could always find a “ton” more!
Appendix
“An Elephant's Anatomy.”, 27 December 2003, ElephantHills, YouTube, https://www.youtube.com/watch?v=ICcl5EEU6Ec .
“Mystery of Elephant Infrasounds Revealed | Animal Super Senses.”, 27 November 2015, BBC Earth, YouTube, https://www.youtube.com/watch?v=uQfDazQ9Rkg.
“Studying Elephant Communication.”, 16 February 2017, biointeractive, YouTube, https://www.youtube.com/watch?v=nII9yngRuac.
“What's Inside An Elephant Trunk?.”, 17 January 2019, Science Insider, YouTube, https://www.youtube.com/watch?v=DjpRgi-73bU.
References
3D-Horse. (n.d.). Elephant Animal Leg Bones 3D Model. Retrieved from https://www.cgtrader.com/3d-models/animals/mammal/elephant-animal-leg-bones
Corner, A. (n.d.). Elephant Anatomy. Retrieved from https://animalcorner.org/elephant-anatomy/
de Silva, S., & Wittemyer, G. (2012). A Comparison of Social Organization in Asian Elephants and African Savannah Elephants. International Journal of Primatology, 33(5), 1125-1141. doi:10.1007/s10764-011-9564-1
Detective, A. W. (n.d.). African elephant Retrieved from https://www.africa-wildlife-detective.com/african-elephant.html
Eaden, T. G. o. (n.d.). WHAT IS THE DIFFERENCE BETWEEN AFRICAN AND INDIAN ELEPHANTS? Retrieved from https://gardenofeaden.blogspot.com/2011/06/what-is-difference-between-african-and.html
ElephantVoices. (n.d.-a). Acoustic communication. Retrieved from https://www.elephantvoices.org/elephant-communication/acoustic-communication.html
ElephantVoices. (n.d.-b). Seismic communication. Retrieved from https://www.elephantvoices.org/elephant-communication/seismic-communication.html
Encyclopedia, S. (n.d.). Elephant. In Science Encyclopedia Web Solutions LLC. Retrieved from https://science.jrank.org/pages/2427/Elephant.html
Genin, J. J., Willems, P. A., Cavagna, G. A., Lair, R., & Heglund, N. C. (2010). Biomechanics of locomotion in Asian elephants. Journal of Experimental Biology, 213(5), 694-706. doi:10.1242/jeb.035436
Hutchinson, J. R., Delmer, C., Miller, C. E., Hildebrandt, T., Pitsillides, A. A., & Boyde, A. (2011). From Flat Foot to Fat Foot: Structure, Ontogeny, Function, and Evolution of Elephant “Sixth Toes”. Science, 334(6063), 1699-1703. Retrieved from https://science.sciencemag.org/content/sci/334/6063/1699.full.pdf
Hutchinson, J. R., Famini, D., Lair, R., & Kram, R. (2003). Are fast-moving elephants really running? Nature, 422(6931), 493-494. doi:10.1038/422493a
Hutchinson, J. R., Schwerda, D., Famini, D. J., Dale, R. H., Fischer, M. S., & Kram, R. (2006). The locomotor kinematics of Asian and African elephants: changes with speed and size. Journal of Experimental Biology, 209(Pt 19), 3812-3827. doi:10.1242/jeb.02443
Inc., Pearson E. (2008). Homologous Structures. Retrieved from http://evolutionatccms.weebly.com/uploads/1/1/3/5/11358192/659049970.jpg
Institute, S. s. N. Z. a. C. B. (n.d.). Asian elephant Retrieved from https://nationalzoo.si.edu/animals/asian-elephant
Miller, C. E., Basu, C., Fritsch, G., Hildebrandt, T., & Hutchinson, J. R. (2008). Ontogenetic scaling of foot musculoskeletal anatomy in elephants. Journal of The Royal Society Interface, 5(21), 465-475. doi:doi:10.1098/rsif.2007.1220
O'Connell-Rodwell, C. E. (2007). Keeping an “ear” to the ground: seismic communication in elephants. Physiology (Bethesda, Md.), 22, 287-294. doi:10.1152/physiol.00008.2007
O'Connell, C., Hart, L. A., & Arnason, B. T. (1999). Comments on “Elephant hearing” [J. Acoust. Soc. Am. 104, 1122-1123 (1998)]. Journal of the Acoustical Society of America, 105(3), 2051-2052. doi:10.1121/1.426748
Ramsay, E. C., & Henry, R. W. (2001). Anatomy of the Elephant Foot. In B. Csuti, E. Sargent, & U. Beche (Eds.), The Elephant's Foot: Prevention and Care of Foot Conditions in Captive Asian and African Elephants (pp. 9-12).
Remmers, W., Gameiro, J., Schaberl, I., & Clausnitzer, V. (2017). Elephant (Loxodonta africana) footprints as habitat for aquatic macroinvertebrate communities in Kibale National Park, south-west Uganda. African Journal of Ecology, 55(3), 342-351. doi:https://doi.org/10.1111/aje.12358
Ren, L., Butler, M., Miller, C., Paxton, H., Schwerda, D., Fischer, M. S., & Hutchinson, J. R. (2008). The movements of limb segments and joints during locomotion in African and Asian elephants. Journal of Experimental Biology, 211(Pt 17), 2735-2751. doi:10.1242/jeb.018820
Ren, L., Miller, C. E., Lair, R., & Hutchinson, J. R. (2010). Integration of biomechanical compliance, leverage, and power in elephant limbs. Proceedings of the National Academy of Sciences, 200911396. Retrieved from https://www.pnas.org/content/pnas/early/2010/03/16/0911396107.full.pdf
Tefera, M. (2012). Kinematics and Comparative Anatomy of Some Limb Bones of the African Elephant (Loxodonta africana) and Large Domestic Animals. Journal of Veterinary Anatomy, 5(2), 15-31. doi:10.21608/jva.2012.44872
VA, J. (1998). Panic in elephants during an earthquake. J Bombay Natural Hist Soc, 26.
Valiveti, N. (2018). Why do high frequency radio signals have shorter range?. Retrieved from https://www.quora.com/Why-do-high-frequency-radio-signals-have-shorter-range
Varma, S., Dang, N. X., Van Thanh, T., & Sukumar, R. (2008). The Asian elephants Elephas maximus of Cat Tien National Park, Vietnam: status and conservation of a vanishing population. Oryx, 42(1), 92-99. doi:10.1017/S0030605308010090
Weissengruber, G. E., Egger, G. F., Hutchinson, J. R., Groenewald, H. B., Elsässer, L., Famini, D., & Forstenpointner, G. (2006). The structure of the cushions in the feet of African elephants (Loxodonta africana). Journal of Anatomy, 209(6), 781-792. doi:10.1111/j.1469-7580.2006.00648.x
Weissengruber, G. E., & Forstenpointner, G. (2004). Musculature of the crus and pes of the African elephant (Loxodonta africana): insight into semiplantigrade limb architecture. Anatomy and Embryology, 208(6), 451-461. doi:10.1007/s00429-004-0406-1
Weissengruber, G. E., Fuss, F. K., Egger, G., Stanek, G., Hittmair, K. M., & Forstenpointner, G. (2006). The elephant knee joint: morphological and biomechanical considerations. Journal of Anatomy, 208(1), 59-72. doi:https://doi.org/10.1111/j.1469-7580.2006.00508.x
Western, D., Moss, C., & Georgiadis, N. (1983). Age Estimation and Population Age Structure of Elephants from Footprint Dimensions. The Journal of Wildlife Management, 47(4), 1192-1197. doi:10.2307/3808191
Wikipedia. (n.d.). Muscular hydrostat. In Wikipedia. Retrieved from https://en.wikipedia.org/wiki/Muscular_hydrostat
Wu, J., Zhao, Y., Zhang, Y., Shumate, D., Braccini Slade, S., Franklin, S. V., & Hu, D. L. (2018). Elephant trunks form joints to squeeze together small objects. J R Soc Interface, 15(147). doi:10.1098/rsif.2018.0377
WWF. (n.d.). What's the Difference between Asian and African Elephants? And 8 Other Elephant Facts. Retrieved from https://www.worldwildlife.org/stories/what-s-the-difference-between-asian-and-african-elephants-and-8-other-elephant-facts
Yang, J., Pitarch, E. P., Potratz, J., Beck, S., & Abdel-Malek, K. (2006). Synthesis and analysis of a flexible elephant trunk robot. Advanced Robotics, 20(6), 631-659. doi:10.1163/156855306777361631