Marvels of Eggshell Pigmentation: Why Eggshells Vary so Greatly in Color and Pattern
Leticia Le Goff, Ula Mastej, Jake Pringle, Ryan Romero
Introduction
In contrast to their closest modern relatives, the family Crocodylinae, avian eggs dramatically range in egg size, shape and color. Primarily for protection from predators, many bird species have evolved unique phenotypes including colored shell markings that represent a novel development in the phylum Chordata's gene pool. Selection for egg color became relevant to the avian species when they switched from burying their eggs to building open nests for egg fostering. This selection would have been primordial in the increase of survival of the egg as they became visible to the wider environment, including predators and parasites. Analysis of eggshell pigmentation's effect on camouflaging are presented to highlight the species' adaptive fitness model. As well, chemoprotection and structural reinforcement discussions further the notion that pigmentation directly functions to increase egg survival. Furthermore, the two main pigments of eggshells will be examined along with how the expression of these two can affect architectural characteristics such as egg strength and hatchability. The operation of how these pigments interact with light and how they are selected in co-evolution and due to environmental factors is then discussed. Finally, a comparison between two eggs will highlight how variations of the same functions will result in eggshells of vastly different pigmentation, both between and within species. The Great Tit and the Common Cuckoo will both serve as examples of how genetic inheritance, nesting ecology, environmental conditions and, in the case of the Great Tit, sexual signals influence shell coloration and patterning.
Function
Avian eggshell pigmentation and the associated chemical basis behind it represent an idol case study of class' phenotypic plasticity. With focal points including protection from predators, the environment, and unique biospheres, eggshell pigmentation's function is mostly attributed to improving the fitness of avian species. Ranging from background matching to disruptive coloration, the camouflaging of eggshells is one of the first steps in birds' lifespan ensuring their survival (Mayani-Parás et al., 2015); unsurprisingly, efforts to explain fitness as it relates to the function of eggshell pigmentation are vast. Analysis of the fitness associated with phenotypes, brood parasitism, chemoprotection, and structural strength highlight the selection towards specific pigmentations, patterns and cuticle compositions, and speckledness in avian eggs.
Crypsis
Brood Parasitism's Relationship with Eggshell Camouflaging
Coevolution between various avian egg species has been observed due to relationships of pattern, color, and luminance of egg pigments. Within the avian class, there exists a form of mutualistic relationship between species known as brood parasitism. As described by its name, parents will nest their eggs into other species' habitats in the aim that these designated host parents will foster them, devoting resources, time, and energy into bringing up this chick which is not their own. This allows the original species to gain in fitness without any significant expenditure to itself. The biochemistry behind eggshell qualities serves a pivotal function in this parasitic exchange, both from the original and host parents' perspectives. Visual cues resulting from the chemical background of eggshell pigmentation are found to be one of the most important factors in whether the host parents will reject the egg nested to them (Spottiswoode and Stevens, “Visual modeling shows that avian host parents use multiple visual cues”). If the egg is not properly camouflaged to be one of the host parents' own, rejection of the egg ensues and the cost to the original species is grave. Therefore, guaranteeing said qualities are of correct mimicry is essential and it is this selection/rejection that has caused the aforementioned coevolution between brood parasitic birds and host parents (Spottiswoode and Stevens, “Visual modeling shows that avian host parents use multiple visual cues”). In analyzing the response of host parents to parasitic eggs, a third of rejection was identified to be stimulated by color and pattern variations (Spottiswoode and Stevens, “Visual modeling shows that avian host parents use multiple visual cues”). Such findings indicate that for improved fitness, eggshell qualities of optimum crypsis are selected for by the success of brood parasitism (Gosler et al., “Protoporphyrin and Eggshell Strength”). In well-lit situations, color is the primary visual cue for rejection behaviors, yet in most cases this is not guaranteed and so it is the combination of color with marking sizes, their associated distributions, dominance, and luminance, that allows for host parents ability of an “adaptive defense” (Spottiswoode and Stevens, “Visual modeling shows that avian host parents use multiple visual cues”). From just noticeable difference (jnd) calculations, these final variables (excluding luminance) were outlined as being the primary predictors for variations in rejection (Spottiswoode and Stevens, “Visual modeling shows that avian host parents use multiple visual cues”). Brood parasitism is therefore a first indication that the biochemistry of avian eggshells plays a fundamental role in optimizing the success of egg survival.
Soiling Camouflage
Another take on the discussion of interspecies crypsis is eggshell camouflaging to its surrounding environment. It is necessary for egg survival that shells be properly conspicuous to predators. Therefore, specific characteristics of an avian egg's pigmentation are selected for by the environment they seek to be disguised in – under the assumption that selection manages the genetic array of pigment and pattern distributions among avian eggshells (Hargitai et al., “Biliverdin- and protoporphyrin-based eggshell pigmentation”). Particularly, sticking of environment soil, foliage, etc. to the eggshell and grace of eggshell cuticle chemical components give rise to a form of camouflaging known as soiling (Mayani-Parás et al., 2015). As the shell is therefore subjected to crypsis specific to the environment in which it is found, such a technique has proven successful regardless of original shell phenotype. The prey of gulls upon booby eggs provides evidence of such success, showing that soiling reduces the destruction of booby eggs to a statistical degree of P = 0.001. Not only in terms of destruction, taking of booby eggs was also statistically reduced by P = 0.003 for booby eggs that were soiled independent of their environment in which they were camouflaged – forest versus grassland (Mayani-Parás et al., 2015). Linear and quadratic relations between soiling levels and egg age were also found to a maximum statistical significance of P = 0.0001, indicating a more behavioral based soiling that allows for camouflaging to properly become apparent around the fourth day of incubation (Mayani-Parás et al., 2015). This reasoning is conditional to parental habits and their nurturing of eggs, yet beyond this, Fig. 1 demonstrates the payoff of such soiling. A statistically significant prey decrease of degree P < 0.001 over the first 15 days outlined no more than 20 % of eggs were taken in a given five day interval past this initial incubation period, compared to the original 47 % taking of booby eggs (Mayani-Parás et al., 2015). This then adds confirmation that soiling plays fundamental roles in egg survival. Although phenotypes derived from eggshell genetics may serve sexual selection purposes – a traditional, commonly accepted theory relating female exhibitions of it and its eggs' phenotypes to male mates (Hargitai et al., “Biliverdin- and protoporphyrin-based eggshell pigmentation”) – soiling and its time dependent nature unite that avian eggshell chemical compositions promote species fitness via interspecies crypsis.
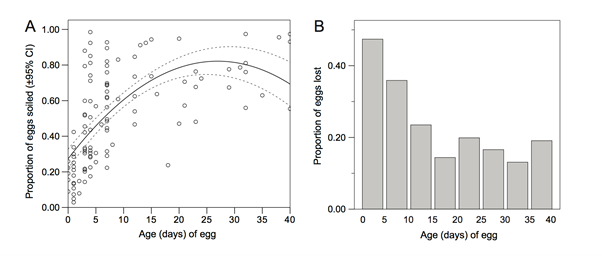
Protection
Chemoprotection Specified to Selective UV-Reflection
It is also found that cuticle patterns and speckledness that result from biochemical compositions of avian eggshells serve the roles of chemoprotection and mechanical support for egg survival. Beginning with the former, it is previously known that organic molecules in outer eggshell layers absorb UV light. The extent to which they do so has formerly been considered a result of the approximately 10 % increased eggshell thickness for speckled areas, a matter of pigment composition (Orlowski et al., 2017). Yet, the comparison of pigmented versus unpigmented eggshells outlines that regardless of whether or not the two main pigments of avian eggshells – biliverdin and protoporphyrin – are present in a species eggs, variation in UV-reflectance still occurs and therefore is a property of other chemical interactions throughout the eggshell surface (Fecheyr-Lippens et al., 2015). Acid – such as disodium ethylenediaminetetraacetic acid – applied to a range of species' eggshells results in cuticle degradation, in turn showing an increase in UV intensity on the embryo. Therefore, UV-reflectance variation between species is deemed an attribute to the predominantly calcium phosphate composition of eggshell cuticles as well as the calcite egg matrix (Fecheyr-Lippens et al., 2015).
Embryo health is then directly related to this chemical identity of avian eggshells. As the roughly 96 % calcite composition of eggshells varies in crystalline structure and chemical dissolution across species, UV light that is selectively absorbed is said to hold effects on camouflaging and mating (Fecheyr-Lippens et al., 2015). This newly proposed mechanism of UV-protection, deemed “structural coloration”, and its related function to sun-exposure protection has inspired man-made textiles that biomime avian eggshell cuticles (Fecheyr-Lippens et al., 2015). Unfortunately, few concrete studies exist to further explain the function of this selective absorption (Fecheyr-Lippens et al., 2015), primarily because researching these effects from the perspective of a bird – receptive to UV light – involves methods that although complex are often inaccurate (Spottiswoode and Stevens, “Visual modeling shows that avian host parents use multiple visual cues”). Proposed effects include the development of embryos based on the quantity of UV light absorbed. For longer incubation periods, an associated reduction in UV absorption was found that could indicate UV rays harmful to embryonic development as they potentially increase the rate of embryonic development (Maurer et al., “First light for avian embryos”). On one part, this provides a potential explanation for the observed selection for paler eggs in low lit environments, an ecological-chemical relationship. On the counter, it leaves uncertainty as to how the calcite matrix of avian eggshells accounts for the harm imposed for longer incubated embryos, as the organic matrix is taken from for skeletal growth (Maurer et al., “First light for avian embryos”). Altogether, the chemical composition of the cuticle and organic matrix of avian eggs serve as a primary UV light regulating mechanism, proposing notions of a chemoprotection function mostly related to embryo health that requires further investigation.
Structural Reinforcement
Whilst extensive research has been provided to explain pigmentation and avian eggshell chemical compositions from a visual function perspective, it is also noted that pigmentation is now being explored for its structural functions. In comparing the fracture resistance and eggshell brittleness of pigmented versus unpigmented eggshells, it has been summarized that avian eggshell pigmentation compensates for thinned shells. Statistics from Great Tit eggs show that unpigmented eggs are 0.006 mm thinner than pigmented eggs to a variation of 0.009 mm (Gosler et al., “Protoporphyrin and Eggshell Strength”). From this, General Linear Mixed Model (GLMM) analysis of cut sections of eggshell showed that in the case of eggshell thinning, sections of pigment provided the most significant structural reinforcement (Gosler et al., “Protoporphyrin and Eggshell Strength”). Such models then infer that this may be a property of the noticeable concentrations of calcium and magnesium found in eggshell areas speckled with pigment (Orlowski et al., 2017). Noting such inferences of pigment distribution, t-test analysis showed shell toughness to have a significant relationship to the intensity of pigmentation (Gosler et al., “Protoporphyrin and Eggshell Strength”). Accordingly, it is seen that the chemistry of avian eggs in part corresponds to specific eggshell maculation for localized structural support.
Such discussions pin aforementioned mimicry as “conventional” reasonings for eggshell pigmentation and turn to the properties of pigmentation for establishing their function in the avian egg (Gosler et al., “Protoporphyrin and Eggshell Strength'). Correlational evidence between pigment size, distribution, and intensity show a spotted ring known as the corona ring to be commonly present around the pole of avian eggs. GLMM then proves the commonly proposed hypothesis that such protoporphyrin concentrations improve fracture toughness on standardized avian eggshell models (Gosler et al., “Protoporphyrin and Eggshell Strength”). Conversely, it is necessary for further experimentation to relate the function of eggshell pigmentation to total eggshell strength; above GLMMs indicate isolated increase in toughness yet provide few quantitative evidence on pigments overall consequences.
Architecture
When thinking about avian eggshell pigmentation and color, it is likely to forget just how different every egg can appear in the avian bird species. For example, the oystercatcher (Haematopus ostralegus) has light beige with dark brown maculations whereas eggs from emus (Dromaius novaehollandiae) are an intensive bluish-green. Knowing that eggs can choose from this wide palette of colors, studying how these colors are obtained and how pigmentation of the egg shell can influence other factors like egg shell strength and hatchability will provide more knowledge regarding the close bond between egg color and pattern.
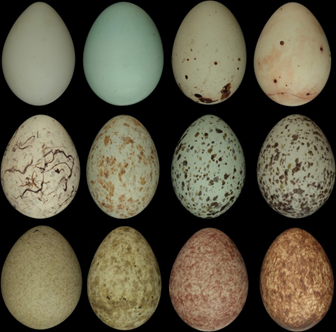
Pigmentation Acquisition and Expression
Color and maculations of the egg are determined during the 24 hours in which the egg is inside the mother hen. About 4 hours after its release from the ovary, the egg, which has been fertilized and through the process of enclosing the membrane over the yolk and albumen, moves through the final parts of the oviduct and reaches the shell gland pouch. The next 20 hours are dedicated to the eggshell biomineralization process where a carbonate shell is deposited and indicates the end of the shell formation. Calcite crystals interlaced with pores surround the egg giving it the hardened structure of the egg. Finally, a thin organic cuticle covers the outer surface of the egg and will exhibit the color and maculation of the egg (Kilner, 2006).
The pigments responsible for both the color and patterning of the egg are deposited in the 4 hours preceding egg-laying and therefore reside primarily in the outer part of the shell and in its cuticle. In fact, pigmentation is emitted by uterine epithelial cells which means that pigmentation is present in uterus fluid and will only manifest on the egg at that stage. The main proteins at the origin of eggshell pigmentation are Protoporphyrin IX and biliverdin IX and its zinc chelate. Each protein has a specific role in deciding the pigmentation of the eggshell and each is regulated by estradiol and progesterone so as to not interfere with each other and create a very different color (Liu et al., 2010). It is important to note that whilst pigmentation may mainly be used to better protect the eggs from external dangers, it may also serve a structural function and impact the role, strength and flexibility of the shell.
Pigmentation Proteins
Protoporphyrin IX and biliverdin IX are two solid organic compounds that are found in eggshell structures and who are strong in color and establish the diversity in egg colors. Studies have shown that Protoporphyrin IX is mainly associated with brown and black pigments, whereas biliverdin IX and its zinc chelate are responsible for white, blues and greens (Ishikawa et al., 2009).Both derive from the heme cycle, a process necessary to bind oxygen in the bloodstream. These proteins are part of the hemoglobin family and use these characteristics so that their biosynthesis takes place in the shell gland, where the egg forms (Wiemann et al., 2017). Studies have shown that eggshell protoporphyrin and biliverdin have their biosynthesis mechanism in the shell gland of the mother hen (Zhao et al., 2006). Remarkably, the two pigments are under independent genetic control, suggesting that either one, both or neither can occur which only adds to the infinite color results available (Jagannath et al., 2008). Many species show a biliverdin based ground color with protoporphyrin speckles.
Biliverdin (BV) and Its Characteristics
As mentioned before, Biliverdin is a green pigment and it is a derivative of hemoglobin breakdown. It eventually displays a blue or green color in the eggshell and mainly coordinates the whole eggshell forming a background color for the egg (Kilner, 2006). The formation of biliverdin happens when the heme group in hemoglobin cleaves at its methane bridge constituent. What is left is the biliverdin which is then reduced to bilirubin, a yellow pigment, by the biliverdin reductase enzyme (“Biliverdin (BV) Isomers Analysis service” | Creative Proteomics, n.d.). Extraordinarily, this reaction can be observed when a bruise turns from deep purple to green-yellow over time.
Protoporphyrin (PP)
In contrast to biliverdin, protoporphyrins will give eggshells colors ranging from yellow to reds and browns (Kilner, 2006). Protoporphyrin IX belongs to the hemoglobin family and consists of four pyrrole rings connected by methane groups (Samiullah et al., 2015). The pigment is deposited onto all shell layers including the shell membrane but the concentration is highest in the outermost layer: the cuticle. Protoporphyrin is mostly localized in spots and speckles across the eggshell but can also form a ground color white or brown like in chicken eggs (“Biliverdin (BV) Isomers Analysis service” | Creative Proteomics, n.d.).
Consequences of Eggshell Pigmentation on Eggshell
When thinking about pigmentation in avian eggshells, the primary explanation is to make the eggs camouflaged. However, it does not explain why some eggs are bright blue and green. In reality, it is not the only reason why there is such a wide variety of shell colors. Eggshell colors can also be associated with shell strength, temperature regulation and hatchability (Ishikawa et al., 2009). Looking at chicken eggs, the nature of eggshell color is still extensively researched. How come some hens lay brown eggs whilst others lay white? Studies have found that the dominant pigment for brown laid eggs is protoporphyrin IX and that this also influences the capacity of shell strength (Lang and Wells, 1987). But in general for all eggs, recent hypothesis suggest that the result of eggshell color are influenced by sex linked genes and other specificities regarding the mother hen (Ishikawa et al., 2009). These colors may be signals directed to the mates indicating the phenotypic qualities of the female in order to increase the paternal care of the chicks (Moreno et al., 2005). It has been noted that the level of biliverdin in the eggshell may signal antioxidant capacity during the laying period. At this stage, progesterone can impact the stress level of the mother hen which in turn influences the functioning of the immune system of the female. During laying, the action of progesterone may induce a higher oxidative stress in females, so females who have a strong immune system can better manage this oxidative stress and may express this throughout the chicks eggshell without contracting any dangerous consequences.
In a sense, it is completely coherent that studies have shown old-age females to lay lighter (i.e., less colored) eggs and that young-age females who have a higher immunocompetence to lay darker and more pigmented eggs (Moreno et al., 2005).
Consequences of Eggshell Pigmentation on Eggshell Strength
One factor that eggshell pigmentation can influence is shell strength. As mentioned before, brown chicken eggs which have high concentration levels in the protoporphyrin pigment have shown to be more resistant in shell strength. Nonetheless, in most eggshells, protoporphyrin manifests in maculations rather than a ground color. It has been noted that these pigment spots demarcated a reduction in eggshell thickness compared to spots with paler pigmentation. Having a fluctuation in eggshell thickness is not abnormal as it may be due to a functional reason. For instance, the crown of the egg is much thinner thus enabling easier hatching for the chickling, but in this case the difference of thickness is due to a calcium deficiency (Gosler et al., “Why are birds' eggs speckled?”). To sum it up, variation in pigmentation is related to variation in shell thickness in egg clutches.
Taking a closer look at eggs laid by Great Tits (Parus Major), all eggs have the presence of dark pigmented maculations on the eggshell. Studies led in nesting grounds of Great Tits revealed that protoporphyrin spots on the eggs are proportionate to shell thickness which varied with calcium availability (Jagannath et al., 2007). When there is a decrease in calcium, there is an increase of fluctuation in the shell thickness and protoporphyrin is deposited on the precise area of shell thinning. Accordingly, eggs that are in low-level calcium areas are more heavily spotted than eggs in high-level calcium (Jagannath et al., 2007). Among eggs, thinner shells are marked by the addition of pigment so it is possible to determine visually how thin a shell is by analyzing the darkness of pigmentation in a specific area.
Not taking into account pigmentation, eggshells are thinner around the shoulder of the egg. This directly influences the shape of the egg and suggests a design constraint since it is found to be true in all eggs. It is also because this is the region where hatching occurs. Hence, the properties of protoporphyrin that strengthen the shell on the outside can also weaken the shell from the inside, thus facilitating the breaking of the shell for the chick when the time comes (Jagannath et al., 2007). The architectural significance of protoporphyrin spots indicates that the bird is adapting to a low calcium environment by adding this pigment within the internal crystalline matrix of the shell.
Operation
Due to the immense and diverse range of functions of eggshell pigmentation, the key to the operation of eggshell pigment is finding a proper balance of colors and patterns to best fulfill these functions. An eggshell must have a color palette that allows it to protect the egg from harmful rays, regulate heat, and make the eggs identifiable to the parents but hidden from predators or similar to another bird's eggs for successful parasitism, so long as these criterions apply to the safety of the egg in question. How the eggshell achieves these functions and balances them in its operation will be examined in the following section.
Interactions of Light and The Eggshell Pigment
Thermoregulation
One of the key functions of an eggshell is to keep optimum temperature within an egg, via the process of thermoregulation. Changing the temperature within an egg by just a few degrees from its optimal incubation values can injure or even kill the egg embryo (Bakken et al., 1978). Thus, thermoregulation requires that the egg absorbs heat and keeps it, if it is in colder climates, or avoids absorption in hotter and more sunlit climates.
In a study completed by David Lahti and Daniel Arida, it was found that the interior of an egg does in fact heat as it is exposed to IR radiation. They found that thermocouples placed a distance away from an IR lamp heated faster if they were within an egg than if they were in the open air (Lahti and Arida, 2016). It was further shown that the degree to which this occurs is dependent upon eggshell pigmentation. One phenomenon that occurs is the “parasol effect”: more intense coloration blocks light transmittance into the egg, causing a smaller percentage of IR waves to pass through it. However, this also means that it is less likely for the light to escape from the egg. This leads to a second phenomenon, the “dark car effect”: the darker an eggshell is, the more light it retains or absorbs. As a consequence of absorbing these IR waves, it retains them as heat (Lahti and Arida, 2016). If they were to just be transmitted or reflected, as in the case of brighter eggs, heat gain would be minimal. Thus the pigmentation of an egg plays a key role in IR wave and heat absorption.
The pigmentation specific to eggshells is incredibly important to understanding the extent of this effect. The desire for camouflage so as to avoid predators suggests that it is optimal for eggs to be colored, in order to blend in with their surroundings. Nevertheless, if pigmentation leads to higher heat retainment, this can be equally catastrophic for the bird embryo to be within a pigmented shell. This apparent contradiction can be resolved with the use of pigments which are reflective of IR radiation. Eggshell pigments, such as protoporphyrin and the biliverdin, reflect about 90 % of incident near-IR waves, even those that result in dark brown spots. Like melanin, these pigments absorb some visible light but are more reflective of IR radiation by 31 – 38 % compared to melanin (Bakken et al., 1978). These absorption characteristics of protoporphyrin and biliverdin thus allow for crypsis of eggs with a smaller heating penalty than the use of melanin as pigment P.
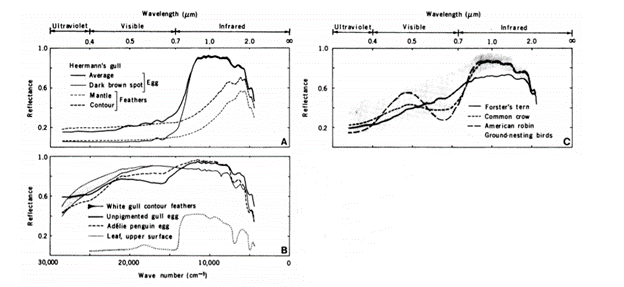
Photoprotection aims to protect the egg's interior from harmful UV rays. Radiation from the UV-B spectrum (290-320 nm) is particularly harmful to bird embryos as it can cause mutations in the DNA sequence early on in development, which will be replicated throughout mitosis. This could lead to growth deformities or even death, especially if it occurs for long durations. Many organisms have adapted to this by incorporating pigments containing conjugate bonds into the tissue they desire to protect, such as melanin or carotenoids, and protoporphyrin and biliverdin in the case of avian eggs (Maurer et al., “Review: an embryo's eye view of avian eggshell pigmentation').
The incident UVB rays are likely subject to more effects than IR rays. The “parasol effect” also occurs, causing eggshells with lower brightness to allow a greater transmission amount of UVB rays, although this trend is not as strong as with IR rays (Lahti et al., 2016) (Fig. 4). The importance of pigment in reflecting UVB rays is also likely correlated with eggshell thickness, as thinner eggshells allow greater UV transmission (Larena and Villar, 2001). An example of the relationship between UVB protection and pigment is shown with Lahti's study on village weavers Ploceus cucullatus. These weavers were introduced into a habitat without their brood parasite and as a result variation between eggs of the Ploceus cucullatus declined, causing an increase in blue pigmentation, which in turn reflects more UVB rays (Maurer et al., “Review: an embryo's eye view of avian eggshell pigmentation”). Removing the pressure of egg variation due to the brood parasite appears to have pushed the selective pressure more towards sunlight protection, as was consistent with their new environment.

Further Relationships Between Pigment, Crypsis, Structure and EMR
It is important to note that eggshell pigment is not the sole cause of photoprotection, and neither is it the sole cause of thermoregulation. As mentioned earlier, for photoprotection, the cuticle plays a significant role, as well as the shell structure itself. If the outer layers are removed from a chicken eggshell for example — leaving behind the calcite structure, — its UV reflectance increases. This change also differs across species, suggesting that the nanostructuring of calcite crystals play a role, since no known pigment absorbs light across all wavelengths except UV (Fecheyr-Lippens et al., 2015). Another fascinating phenomenon that occurs when shell structure is unpigmented or weak, is the substitution of shell pigmentation for embryonic skin pigmentation. Many tropical birds have black embryos or hatchlings, suggesting that if the eggshell is not sufficient in its UVB protection, skin pigmentation adds to the protection with the use of melanin (Maurer et al., “Review: an embryo's eye view of avian eggshell pigmentation”). Likewise, thermoregulation is not only dependent on pigmentation but also eggshell thickness, as thicker eggshells tend to retain more heat (Lahti et al., 2016). Thus, the usefulness of pigment in the eggshell for photoprotection and thermoregulation must be considered with various other factors.
Additional factors contributing to the necessity of pigment are whether the eggs are kept in a nest shaded from light or dark crevices. The eggs that are kept this way need not reflect much light, resulting in less pigment. The need for camouflage is also diminished due to either being hidden in the dark – crevices – or covered otherwise with nest elements such as twigs and leaves to be hidden. Moreover, the need for camouflage is further decreased if the parents brood for significant amounts of time. In fact, in such a situation, bright coloring may be used as a signal to parents – typically the male – to be more engaged in brooding or bringing food to the brooding mother, as conspicuous eggs need more protection from predators (Hanley et al., 2010). Thus, how much pigment is needed for the proper operation of thermoregulation and photoprotection depends on both structural elements of the eggshell and embryo as well as the surrounding lighting and environment.
Operation of Mimicry and Parasitism Prevention
Another important function of an eggshell is to serve as an identifier. As previously mentioned, parents use a combination of color with marking sizes, associated distributions, dominance, and luminance to identify their eggs. Additionally, as an incentive for brooding and feeding in some cases, egg recognition and discrimination constitutes a mechanism by which parents can differentiate their eggs from parasitic eggs. Meanwhile, pattern mimicry serves as a mechanism by which parasites aim to ensure the survival of their young.
There are two possible mechanisms used by host parents to ensure the survival of their young and elimination of brood parasites: polymorphism and discrimination. Polymorphism constitutes variation in the egg patterns that a host parent will produce whereas discrimination refers to the rejection of eggs based on characteristics not matching their own common patterns. It has been found that species with greater polymorphism tend to be less discriminatory whilst those that are less polymorphic are more discriminatory. Interestingly, neither greater polymorphism nor greater discrimination constitute a better mechanism for ensuring the survival of their young and elimination of parasites; they simply represent two alternatives (Spottiswoode and Stevens, “How to evade a coevolving brood parasite”).
Since the host parents have mechanisms in place to reject foreign offspring using color variation of eggshells, so too do the brood parasites have mechanisms by which they increase the success of their offspring. This results in the process of coevolution: two or more species evolving continuously to possess similar traits by putting selective pressures on one another.
The operation of these mechanisms is best illustrated with the example of prinia and its brood parasite: the cuckoo finch. In C. Spottiswoode's 40-year study, these two birds have shown an increase in variation of color hue and extremity over time, although the prinia's color variation is greater than that of the cuckoo's (Fig. 5). Furthermore, it was shown that older generations of parasitic eggs are more similar to older versions of host eggs than the current egg generation, and that current parasitic eggs are more similar to those of current host eggs than past host eggs (Spottiswoode and Stevens, “Host-Parasite Arms Races and Rapid Changes in Bird Egg Appearance”). These findings suggest that parasites must also exhibit a high degree of polymorphism to be able to compete with the polymorphism of the host and prevent discrimination leading to the rejection of their young by the host. Thus, the mechanism of polymorphism is useful to hosts and parasites alike to aid in the reproduction of their species, and contributes to the selection of egg color alongside selective pressures related to crypsis, photoprotection and thermoregulation.

Comparative Study of Two Bird Eggs
Two bird eggs will be further analyzed: those of the Parus major, also known as the Great Tit, and the Cuculus canorus, or the Common Cuckoo. For each bird, inheritance patterns, nesting ecology, and environmental conditions, will be discussed as well as how they can potentially play a role in eggshell patterning. Additionally, sexual signaling and how it contributes to shell patterning will be considered in the case of the Great Tit.
Parus major – The Great Tit
The Great Tit is a passerine and a part of the titmouse family. The eggs of this bird are white with red-brown spots (Fig. 6), which are produced by the protoporphyrin pigment (Stoddard et al., “Egg Speckling Patterns Do Not Advertise Offspring Quality”). The reasons for this specific shell patterning can be explained by a combination of evolution and inheritance patterns, nesting ecology, environmental factors, and sexual signaling.
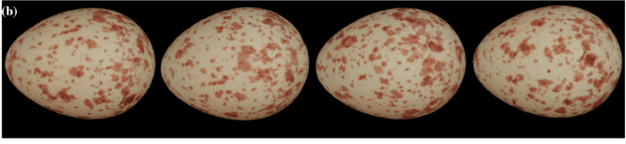
Genetic Inheritance
Firstly, it has been hypothesized that spotting patterns on Great Tit eggs can be genetically inherited, with inheritance through maternal line. When observing Great Tit eggs, it is not uncommon for there to be a great difference in spotting patterns between eggs of different clutches. However, within clutches, spotting tends to be relatively similar. One study conducted on Great Tit clutches laid over a period of eight years noted exceptional female repeatability and negligible male repeatability, showing that female Great Tits tend to lay eggs of similar patterns (Gosler et al., “Inheritance and variation in eggshell patterning in the great tit”). Additionally, when studying the inheritance of spotting patterns throughout a lineage, the daughters' eggs resembled those of their mothers and maternal grandmothers, but not those of their paternal grandmothers (Gosler et al., “Inheritance and variation in eggshell patterning in the great tit').
Given that there are noticeable similarities in shell patterns within clutches and between mother-daughter lines, it is possible to conclude that spotting is unlikely to be a random event. Similarities tended to be found across the maternal side of Great Tit eggs. Thus, any genetic inheritance patterns are likely to be a result of genes on the female sex-specific W chromosome (Gosler et al., “Inheritance and variation in eggshell patterning in the great tit'). Although this does not explain the functional reasons for shells being white with red-brown spots, there is a strong argument of Great Tit patterning being genetically inherited.
Nesting Ecology
Great Tits are classified as hole-nesting birds since their nests are found in holes or cavities and tend to be covered by leaves and other materials (Stoddard et al., “Egg Speckling Patterns Do Not Advertise Offspring Quality”). In comparison, open-nesting birds have to contend with predators and, in those cases, spotting will function as camouflage to provide crypsis to the eggs. Nevertheless, since Great Tits are hole-nesting and cover their nests throughout most of the incubation period, it is unlikely that shell patterns provide camouflage from predators.
As previously explained, in brood-parasitic species, speckling is particularly important as it allows eggs to mimic those of their host and prevent ejection from the host nest (Stoddard et al., “Imperfectly Camouflaged Avian Eggs”). On the flip side, birds that are the target of brood parasitism may also lay maculated eggs as a means of identifying their own eggs in the nest (Cherry and Golser, 2010). While brood parasitism might explain shell maculation in brood parasite-host systems, the Great Tit is neither a brood parasite nor a host. Therefore, it is improbable that speckling is a result of this interaction.
Lastly, there is a relationship between nest ecology and thermal stress, which can explain differences in egg coloration and patterns. During exposure to solar radiation, the yolks of darkly pigmented eggs reach temperatures that are deadly to the developing embryo (Montevecchi, 1976). While dark eggshells were seen to cause overheating, protoporphyrin and biliverdin are thought to have properties that reduce the effects of solar radiation by reflecting light in the infrared range, which has been explained in previous sections of this paper (Stoddard et al., “Imperfectly Camouflaged Avian Eggs”). Neither of these theories explains the shell patterning of the Great Tit. As a cavity-nesting bird that does not get direct sunlight exposure, a darkly pigmented shell would not negatively affect the embryo and might actually prevent chilling. Nonetheless, the Great Tit is eggs are white. Comparatively, if spotting provides protection from solar radiation, Great Tits do not necessarily need this protection as cavity-nesters who are not exposed to high amounts of sunlight.
Environmental Conditions
Next, environmental conditions – such as weather and breeding location density – may have an impact on shell maculation of Great Tits. When Great Tits breed earlier in the season, their eggs usually have darker spots, whereas colder weather and high-density breeding locations will cause more spotting (Hargitai et al., “Effects of environmental conditions on the egg mass, yolk antioxidant level, eggshell thickness and eggshell spotting patterns of Great Tits”). Additionally, when eggs are laid further into the breeding season, spotting coverage was greatly reduced. These results show that food availability – particularly caterpillar availability – might influence spot darkness and that coverage is influenced by temperature and breeding density. It is possible that “adverse environmental circumstances (nutritional and social stress, lower ambient temperature)” affect maculation by increasing protoporphyrin pigmentation (Hargitai et al., “Effects of environmental conditions on the egg mass, yolk antioxidant level, eggshell thickness and eggshell spotting patterns of Great Tits”).
Sexually Selected Eggshell Coloration (SSEC)
Finally, another predicted function of eggshell pigmentation is explained by the sexually selected eggshell coloration hypothesis. This posits that shell patterns and coloration is used to persuade males to contribute more to breeding and to advertise female quality (Stoddard et al., “Egg Speckling Patterns Do Not Advertise Offspring Quality”). However, Stoddard et al. did not find any evidence to support the theory that maculation has any effect on paternal behavior. In this study, there were no indications that males responded in any way to spotting on Great Tit eggs (Stoddard et al., “Egg Speckling Patterns Do Not Advertise Offspring Quality”). One potential explanation for this is that the nest, being a cavity, does not provide enough light for males to accurately see shell patterns. Similarly, eggs are usually kept covered which will also prevent males from seeing the shell clearly (Stoddard et al., “Egg Speckling Patterns Do Not Advertise Offspring Quality”). Hence, maculation on Great Tit eggs is unlikely to serve as a signal to entice males to contribute more in breeding.
The SSEC hypothesis also postulates that shell coloration and patterning is used as a signal to advertise female and offspring quality. As an antioxidant pigment, it is theorized that biliverdin pigmentation acts to showcase the antioxidant capacity of females. Similarly, protoporphyrin may also act as a signal. As a pro-oxidant, protoporphyrin is thought to induce oxidative stress in females. If this is the case, spotted eggs potentially indicate dysfunctions in excreting harmful molecules and signal poor health (Stoddard et al., “Egg Speckling Patterns Do Not Advertise Offspring Quality”). On the other hand, heavy maculation may demonstrate “oxidative tolerance” and the ability to excrete “damaging [protoporphyrin] pigments” (De Coster et al., 2013). One study found a relationship between the distribution of red-brown spots and female antioxidant capacity: females with “high enzymatic-thiolic antioxidant capacity” tended to produce eggs that had a more homogenous distribution of maculation (Giordano et al., 2015). Additionally, a different experiment found a correlation between speckledness and bird condition: heavy Great Tits tended to lay eggs with lighter and less extensive patterns (Stoddard et al., “Egg Speckling Patterns Do Not Advertise Offspring Quality”). Although no definitive explanation for Great Tit shell patterning has been found, there is significant evidence that shell maculation serves to display female's response to oxidative stress, either in a positive or negative manner.
Cuculus canorus – The Common Cuckoo
The Common Cuckoo, also known as the European cuckoo, is part of the family Cuculidae. Common Cuckoos are brood parasites, meaning eggs will be laid in host nests and cuckoos will rely on their hosts to raise their young (“Common Cuckoo” | Audubon Guide to North American Birds | National Audubon Society, n.d.). Shell patterning in cuckoos has therefore evolved to mimic their host's eggs as closely as possible (Fig. 7). Genetic inheritance, nesting conditions, and environmental conditions are all factors contributing to eggshell spotting seen in this bird.
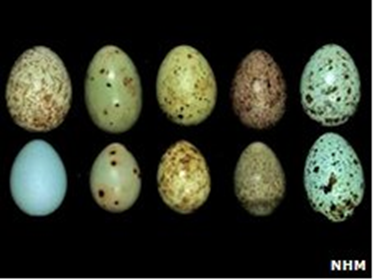
Genetic Inheritance
Cuckoos are divided into gentes – host-specific races – with the female of the races laying eggs that mimic specific hosts. Since each gens is specific to a particular host, it is important that the egg patterning does not vary; else the eggs will not be accepted in the host nest. Due to this, it is theorized that gentes are solely linked to the female lineages, and the males simply maintain the cuckoos as one species (Gibbs et al., 2000). Female cuckoos will lay eggs that match its host eggs with high repeatability in spotting and color. Males, however, are seen cross-mating with females from many different gentes (Fossøy et al., 2011). This supports the idea that genes controlling shell maculation are found on the female-specific W sex chromosome, much like in the Great Tit (Gibbs et al., 2000).
That said, one study on DNA inheritance found “significant genetic differentiation in both biparentally inherited microsatellite DNA and maternally inherited mitochondrial DNA” (Fossøy et al., 2011). This suggests that males might actually contribute to shell maculation. Genes that code for eggshell patterning could possibly be located on autosomal chromosomes, and not sex chromosomes (Fossøy et al., 2011).
Nesting Ecology
As brood parasites, cuckoo eggs must be able to accurately mimic their host eggs, with different gentes of cuckoos specializing in the mimicry of different hosts. As a result, eggshell patterns can vary greatly between cuckoos of differing gentes (Stoddard and Stevens, 2010). Since not all hosts have the same rejection capabilities, patterning is also dependent on whether host birds may recognize and reject cuckoo eggs; species with strong host rejection pushed cuckoos to develop more advanced mimicry (Stoddard and Stevens, 2010). For example, the Great Reed Warbler is the cuckoo's primary host. Consequently, the cuckoo eggs have evolved similar maculations and coloring as Great Reed Warbler eggs (Cherry and Gosler 2010) (Fig. 8). This parasite-host relationship is seen to be rather stable: when data collected over 70 years was studied, “high parasitism rate, high rate to multiple parasitism and mid-level rejection rate […] seem[ed] to be consistent over time”, suggesting that the relation is in “dynamic evolutionary equilibrium” (Geltsch et al., 2017). This highlights the efficiency of this particular egg design from brood parasitism with Great Reed Warblers.
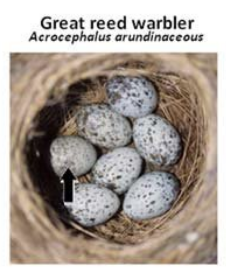
Dunnocks also act as hosts to the common cuckoo. However, in this case, cuckoo eggs have evolved less extensive mimicry. Dunnock eggs are small and pale blue, but cuckoos still continue to lay larger eggs with grey spots (Kirby-Green, n.d.) (Fig. 9). Despite this obvious difference, Dunnocks do not reject cuckoo eggs and accept them in their nests. It is thought that Dunnocks have not yet evolved the necessary defenses and are at an earlier “stage of the coevolutionary arms race” (Stoddard and Stevens, 2010). Thus, this gens' design is still highly effective and does not have to accurately match host coloring or maculation.

Environmental Conditions
Lastly, there is thought to be a link between environmental conditions, specifically temperature and rainfall, on the pigmentation of common cuckoo eggs. If eggshell pigmentation serves as a signal for female quality, temperature and rainfall – which directly impacts food availability – can cause changes in shell coloration (Avilés et al., 2007). One study, conducted by Avilés et al. on the Common Cuckoo and its host the Reed Warbler, showed a correlation between rainfall and cuckoo coloration: “cuckoo eggs were bluer and greener in years with a high relative spring rainfall” (Avilés et al., 2007).
One possible explanation for this is that heavy rainfall and changes in temperature might affect insect availability and thus impact female quality. Additionally, there was a noticeable improvement in cuckoo mimicry during times when heavy rainfall was observed: the intensity of blue-green pigmentation increased during rainy seasons (Avilés et al., 2007). This study proposes that possible signaling function of eggshells and, in the case of the brood parasitic cuckoo, mimicry function may be influenced by temperature and rain patterns and therefore directly eggshell patterning.
Conclusion
Avian eggs pose enormous complexity to ecologists, biologists, physicists, and engineers who attempt to study them. Focus has been drawn to the pigmentation of eggshells across many bird species to outline that whilst the goal off all organisms is increased fitness – whether that be via the function of the many camouflaging techniques of eggshells, chemoprotection, trait expression, structural reinforcement, or thermoregulation and photoprotection – the design of doing so takes on unique distributions, colors, and speckledness, many of which have yet to be analyzed to a full extent. Specifically, discussions ranging from the vibrant blue of Dunnock eggs to the orange speckles of Great Tit samples provide takeaways for engineering principles: biochemistry essential to the survival of any species must be adaptive. The genetic basis of shell pigmentation is significantly dependent on environmental cues and it is this selection for species success in the changing biosphere that promotes avian eggs as one of the most captivating, naturally bioengineered structures to date.
References
Avilés, J. M., Stokke, B. G., Moksnes, A., Røskaft, E., & Møller, A. P. (2007). Environmental conditions influence egg color of reed warblers Acrocephalus scirpaceus and their parasite, the common cuckoo Cuculus canorus. Behavioral Ecology and Sociobiology, 61(3), 475-485. doi:10.1007/s00265-006-0275-0
BAKKEN, G. S., VANDERBILT, V. C., BUTTEMER, W. A., & DAWSON, W. R. (1978). Avian Eggs: Thermoregulatory Value of Very High Near-Infrared Reflectance. Science, 200(4339), 321-323. doi:10.1126/science.200.4339.321
Brennand, E. (2011, Thursday, 24 March 2011). Cuckoo in egg pattern ‘arms race'. Retrieved from http://news.bbc.co.uk/earth/hi/earth_news/newsid_9418000/9418131.stm
Brulez, K., Choudhary, P. K., Maurer, G., Portugal, S. J., Boulton, R. L., Webber, S. L., & Cassey, P. (2014). Visual scoring of eggshell patterns has poor repeatability. Journal of Ornithology, 155(3), 701-706. doi:10.1007/s10336-014-1053-9
Brulez, K., Mikšík, I., Cooney, C. R., Hauber, M. E., Lovell, P. G., Maurer, G., . . . Cassey, P. (2016). Eggshell pigment composition covaries with phylogeny but not with life history or with nesting ecology traits of British passerines. Ecology and Evolution, 6(6), 1637-1645. doi:https://doi.org/10.1002/ece3.1960
CHERRY, M. I., & GOSLER, A. G. (2010). Avian eggshell coloration: new perspectives on adaptive explanations. Biological Journal of the Linnean Society, 100(4), 753-762. doi:10.1111/j.1095-8312.2010.01457.x
De Coster, G., De Neve, L., & Lens, L. (2013). Intra-clutch variation in avian eggshell pigmentation covaries with female quality. Journal of Ornithology, 154(4), 1057-1065. doi:10.1007/s10336-013-0974-z
Fecheyr-Lippens, D. C., Igic, B., D'Alba, L., Hanley, D., Verdes, A., Holford, M., . . . Shawkey, M. D. (2015). The cuticle modulates ultraviolet reflectance of avian eggshells. Biology Open, 4(7), 753-759. doi:10.1242/bio.012211
Fossøy, F., Antonov, A., Moksnes, A., Røskaft, E., Vikan, J. R., Møller, A. P., . . . Stokke, B. G. (2011). Genetic differentiation among sympatric cuckoo host races: males matter. Proceedings of the Royal Society B: Biological Sciences, 278(1712), 1639-1645. doi:doi:10.1098/rspb.2010.2090
Geltsch, N., Moskát, C., Elek, Z., Bán, M., & Stevens, M. (2017). Egg spotting pattern in common cuckoos and their great reed warbler hosts: a century perspective. Biological Journal of the Linnean Society, 121(1), 50-62. doi:10.1093/biolinnean/blw035
Gibbs, H. L., Sorenson, M. D., Marchetti, K., de L. Brooke, M., Davies, N. B., & Nakamura, H. (2000). Genetic evidence for female host-specific races of the common cuckoo. Nature, 407(6801), 183-186. doi:10.1038/35025058
Giordano, M., Costantini, D., Pick, J. L., & Tschirren, B. (2015). Female oxidative status, egg antioxidant protection and eggshell pigmentation: a supplemental feeding experiment in great tits. Behavioral Ecology and Sociobiology, 69(5), 777-785. doi:10.1007/s00265-015-1893-1
Gosler, A. G., Barnett, P. R., & James Reynolds, S. (2000). Inheritance and variation in eggshell patterning in the great tit Parus major. Proceedings of the Royal Society of London. Series B: Biological Sciences, 267(1461), 2469-2473. doi:doi:10.1098/rspb.2000.1307
Gosler, A. G., Connor, O. R., & Bonser, R. H. C. (2011). Protoporphyrin and Eggshell Strength: Preliminary Findings from a Passerine Bird. Avian Biology Research, 4(4), 214-223. doi:10.3184/175815511×13207833399666
Gosler, A. G., Higham, J. P., & James Reynolds, S. (2005). Why are birds' eggs speckled? Ecology Letters, 8(10), 1105-1113. doi:https://doi.org/10.1111/j.1461-0248.2005.00816.x
Hanley, D., Doucet, S. M., & Dearborn, D. C. (2010). A Blackmail Hypothesis for the Evolution of Conspicuous Egg Coloration in Birds. The Auk, 127(2), 453-459. doi:10.1525/auk.2009.09090
Hargitai, R., Boross, N., Nyiri, Z., & Eke, Z. (2016). Biliverdin- and protoporphyrin-based eggshell pigmentation in relation to antioxidant supplementation, female characteristics and egg traits in the canary (Serinus canaria). Behavioral Ecology and Sociobiology, 70(12), 2093-2110. doi:10.1007/s00265-016-2214-z
Hargitai, R., Herényi, M., Nagy, G., Nyiri, Z., Eke, Z., & Török, J. (2016). Effects of environmental conditions on the egg mass, yolk antioxidant level, eggshell thickness and eggshell spotting patterns of Great Tits (Parus major). Journal of Ornithology, 157(4), 995-1006. doi:10.1007/s10336-016-1348-0
Ishikawa, S.-i., Suzuki, K., Fukuda, E., Arihara, K., Yamamoto, Y., Mukai, T., & Itoh, M. (2010). Photodynamic antimicrobial activity of avian eggshell pigments. FEBS Letters, 584(4), 770-774. doi:https://doi.org/10.1016/j.febslet.2009.12.041
Jagannath, A., Shore, R. F., Walker, L. A., Ferns, P. N., & Gosler, A. G. (2008). Eggshell pigmentation indicates pesticide contamination. Journal of Applied Ecology, 45(1), 133-140. doi:https://doi.org/10.1111/j.1365-2664.2007.01386.x
Kaufman, K. (1996). Lives of North American Birds. Boston, MA: Houghton Mifflin
Kilner, R. M. (2006). The evolution of egg colour and patterning in birds. Biological Reviews, 81(3), 383-406. doi:https://doi.org/10.1017/S1464793106007044
Kirby-Green, E. (n.d.). Dunnocks and a Cuckoo in the Nest. Retrieved from https://www.sncv.org.uk/species-spotlight-dunnocks-and-a-cuckoo-in-the-nest/
Lahti, D. C., & Ardia, D. R. (2016). Shedding Light on Bird Egg Color: Pigment as Parasol and the Dark Car Effect. American Naturalist, 187(5), 547-563. doi:10.1086/685780
Lang, M. R., & Wells, J. W. (1987). A Review of eggshell pigmentation. World's Poultry Science Journal, 43(3), 238-246. doi:10.1079/WPS19870016
Larena, A., & Villar, M. A. (2001). Optical properties of CaCO3-filled poly(ethylene-co-vinyl acetate) films. Optical Materials, 17(3), 437-442. doi:10.1016/S0925-3467(01)00063-5
Leonard, P. (2017, 12 June 2017). The Beauty and Biology of Egg Color. Living Bird(Summer 2017). Retrieved from https://www.allaboutbirds.org/news/the-beauty-and-biology-of-egg-color/
Liu, H.-c., Hsiao, M. C., Hu, Y. H., Lee, S. R., & Cheng, W. (2010). Eggshell Pigmentation Study in Blue-shelled and White-shelled Ducks. Asian-Australasian Journal of Animal Sciences, 23. doi:10.5713/ajas.2010.90256
Liu, H. C., Hsiao, M. C., Hu, Y. H., Lee, S. R., & Cheng, W. T. K. (2009). Eggshell Pigmentation Study in Blue-shelled and White-shelled Ducks. Asian-Australas J Anim Sci, 23(2), 162-168. doi:10.5713/ajas.2010.90256
Maurer, G., Portugal, S. J., & Cassey, P. (2011). Review: an embryo's eye view of avian eggshell pigmentation. Journal of Avian Biology, 42(6), 494-504. doi:https://doi.org/10.1111/j.1600-048X.2011.05368.x
Maurer, G., Portugal, S. J., Hauber, M. E., Mikšík, I., Russell, D. G. D., & Cassey, P. (2015). First light for avian embryos: eggshell thickness and pigmentation mediate variation in development and UV exposure in wild bird eggs. Functional Ecology, 29(2), 209-218. doi:https://doi.org/10.1111/1365-2435.12314
Mayani-Parás, F., Kilner, R. M., Stoddard, M. C., Rodríguez, C., & Drummond, H. (2015). Behaviorally Induced Camouflage: A New Mechanism of Avian Egg Protection. The American Naturalist, 186(4), E91-E97. doi:10.1086/682579
Montevecchi, W. A. (1976). Field Experiments on the Adaptive Significance of Avian Eggshell Pigmentation. Behaviour, 58(1/2), 26-39. Retrieved from http://www.jstor.org/stable/4533754
Moreno, J., Morales, J., Lobato, E., Merino, S., Tomás, G., & Martínez-de la Puente, J. (2005). Evidence for the signaling function of egg color in the pied flycatcher Ficedula hypoleuca. Behavioral Ecology, 16(5), 931-937. doi:10.1093/beheco/ari072
Orłowski, G., Pokorny, P., Dobicki, W., Łukaszewicz, E., & Kowalczyk, A. (2017). Speckled and plain regions of avian eggshells differ in maternal deposition of calcium and metals: A hitherto overlooked chemical aspect of egg maculation. The Auk, 134(3), 721-731. doi:10.1642/auk-17-7.1
Proteomics, C. Biliverdin (BV) Isomers Analysis service. Retrieved from https://www.creative-proteomics.com/services/biliverdin-bv-isomers-analysis-service.htm
Samiullah, S., Roberts, J. R., & Chousalkar, K. (2015). Eggshell color in brown-egg laying hens — a review. Poultry Science, 94(10), 2566-2575. doi:10.3382/ps/pev202
Society, N. A. (n.d.). Common Cuckoo. Retrieved from https://www.audubon.org/field-guide/bird/common-cuckoo
Spottiswoode, C. N., & Stevens, M. (2010). Visual modeling shows that avian host parents use multiple visual cues in rejecting parasitic eggs. Proceedings of the National Academy of Sciences, 107(19), 8672. doi:10.1073/pnas.0910486107
Spottiswoode, C. N., & Stevens, M. (2011). How to evade a coevolving brood parasite: egg discrimination versus egg variability as host defences. Proceedings of the Royal Society B: Biological Sciences, 278(1724), 3566-3573. doi:doi:10.1098/rspb.2011.0401
Spottiswoode, C. N., & Stevens, M. (2012). Host-Parasite Arms Races and Rapid Changes in Bird Egg Appearance. The American Naturalist, 179(5), 633-648. doi:10.1086/665031
Stevens, M. (2011). Avian Vision and Egg Colouration: Concepts and Measurements. Avian Biology Research, 4(4), 168-184. doi:10.3184/175815511×13207790177958
Stoddard, M. C., Fayet, A. L., Kilner, R. M., & Hinde, C. A. (2012). Egg Speckling Patterns Do Not Advertise Offspring Quality or Influence Male Provisioning in Great Tits. PloS One, 7(7), e40211. doi:10.1371/journal.pone.0040211
Stoddard, M. C., Marshall, K. L. A., & Kilner, R. M. (2011). Imperfectly Camouflaged Avian Eggs: Artefact or Adaptation? Avian Biology Research, 4(4), 196-213. doi:10.3184/175815511×13207484398647
Stoddard, M. C., & Stevens, M. (2010). Pattern mimicry of host eggs by the common cuckoo, as seen through a bird's eye. Proceedings of the Royal Society B: Biological Sciences, 277(1686), 1387-1393. doi:10.1098/rspb.2009.2018
Stoddard, M. C., & Stevens, M. (2011). AVIAN VISION AND THE EVOLUTION OF EGG COLOR MIMICRY IN THE COMMON CUCKOO. Evolution, 65(7), 2004-2013. doi:https://doi.org/10.1111/j.1558-5646.2011.01262.x
Wiemann, J., Yang, T. R., Sander, P. N., Schneider, M., Engeser, M., Kath-Schorr, S., . . . Sander, P. M. (2017). Dinosaur origin of egg color: oviraptors laid blue-green eggs. PeerJ, 5, e3706. doi:10.7717/peerj.3706 Zhao, R., Xu, G. Y., Liu, Z. Z., Li, J. Y., & Yang, N. (2006). A study on eggshell pigmentation: biliverdin in blue-shelled chickens. Poultry Science, 85(3), 546-549. doi:10.1093/ps/85.3.546