Exploring the Structure and Functionality of Flippers
McKenna Baron, Nour Hanna, Guillaume Rodier, Gabriel Straface
Introduction
Among all living organisms, locomotion is extremely important. For large, multicellular organisms, it might be useful to find more suitable climates, or to escape from predators. For all living things, it is necessary to find nutrition, add diversity to the gene pool and limit inbreeding (Safran and Nosil, 2012). Methods and specialized structures for mobility vary widely between all living things. The following analysis takes a close look at flippers from different animals.
A flipper is a broad limb of an animal which is well adapted for movement through water (“Flipper” | Merriam-Webster Dictionary, n.d.). As a result, flippers are only found in animals that dwell in or near water. This also means that the fins of fish are not considered to be flippers as they are not considered to be limbs per se (“Fin” | Merriam-Webster Dictionary, n.d.).
To begin, the functions of flippers are analyzed. Flippers are interpreted as an application of Newton's third law of motion. To continue, the reason why larger flippers are not necessarily more beneficial is discussed. Then, the significance of the number of flippers and their position is explored. Moreover, the hybridization of the wing and flipper is examined.
The focus of the analysis is the architecture of flippers. To satisfy their new function, the limbs of aquatic mammals have become flippers over the years of evolution. The flippers of various morphologies, including cetaceans, sirenians, pinnipeds, and marine carnivores have different functions that are explored within this analysis (Cooper, 2009). Afterwards, the effect of their design on hydrodynamicity and thermoregulation, along with other purposes such as foraging, is regarded.
Subsequently, the operation of flippers in different animals is examined, including how seals create powerful ‘claps' to propel themselves through water, sharks benefit from the arrangement of their fins that sharpen their agility, and ducks generate thrust by modifying the surface area of their webbed feet as they swim.
Lastly, the structures and functions of flippers and fins are compared among numerous animals. Sharks have various types of fins whose structures and functions are examined, followed by a comparison between the fins and flippers of the dolphin, an aquatic mammal. Finally, the flippers and webbed feet of aquatic birds are considered, specifically those of the penguin. The flippers of these birds carry out a similar purpose to the flippers and fins of fish and aquatic mammals, but differ in specific function, structure, and evolution.
Function of Flippers
The main purpose of flippers is to move through water efficiently. Therefore, they are far better at this goal than other appendages. To illustrate this, we can use the example of penguins, for which millions of years of natural selection have led to the transformation of wings into flippers. Unlike other flightless birds that still have wings but have become too heavy to fly, penguins have simply lost their wings completely, replaced by true flippers, which explains why they are unable to fly (Handwerk, 2013).
To generate forward movement in water, flippers take advantage of Sir Isaac Newton's Third Law of Motion, which states that “All forces occur in pairs, and these two forces are equal in magnitude and opposite in direction”. This means that an animal must displace water in the opposite direction to their desired swimming direction. In turn, the water will also displace them, propelling them forward with a force proportional to the amount displaced. This suggests that the efficacy of a flipper depends partly on the amount of water it can displace, which is influenced by whether its surface is watertight or not (water must be displaced in such a way that it does not pass through the flipper), its range of motion, and its surface area. This can lead to the misconception that bigger is better, even though the proportions of flipper size relative to the animal's size remain similar among all animals with flippers.
While it is true that larger flippers would indeed lead to more water displacement and improve overall locomotion, a slew of problems may arise: fine movements would become harder, leading the animal to be less nimble, and drag would become more prominent, to the point where it would be counterproductive. An example of this is whales who, being large animals, have very big flippers. Due to their size, whales are not particularly agile, yet the effect of drag from their flippers is rarely considered. To minimize this drag, whales have developed several adaptations, including bumps on their flippers to improve their hydrodynamics (Cooper, 2009; Fish et al., “The Tubercles on Humpback Whales' Flippers”; Ashley, 2004). This adaptation helps the whale be more efficient at both accelerating and cruising, and it conserves energy, minimizing the necessary food intake, which for a whale is already very large (up to 1 469 kg of food daily for an average blue whale) (Brodie, 1975).
Furthermore, the efficiency of flippers, during locomotion or cruising, is influenced by their quantity and position. A study done by a team of researchers at the Department of Biology of the Vassar College proved that having four flippers was more efficient at accelerating, but had no effect on top speed, while doubling the energy cost of cruising. Therefore, few aquatic animals have four flippers (Long et al., 2006). Instead, many animals, such as whales, benefit significantly from a tail, which is more efficient at propulsion while consuming less energy. Also, animals which live on both land and water may have feet. This includes, but is not limited to, ducks and other aquatic birds as well as frogs and other amphibians. Many of these animals have webbed feet with functionality similar to flippers (refer to the section Operation of Flippers and their Required Function of this paper for more information).
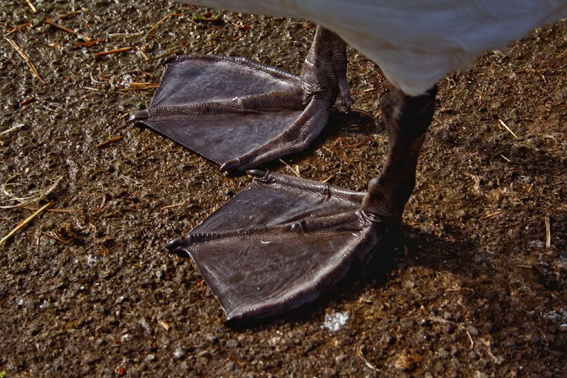
For animals such as seals and sea lions, mobility on sand or snow is also important. Seals and other pinnipeds move on land similarly to how a human crawls while prone. In this case, the flippers must maximize their grip on these surfaces while maintaining a slick aquadynamic profile. This is hard to achieve as grip depends on maximizing friction with the sand and snow but, in most cases, this would also lead to more friction in the water. However, these animals have struck a balance and their flippers are at the most efficient point possible when considering both scenarios (Tennett et al., 2018).
Aquatic and diving birds, with penguins being an exception, have hybridized the wing and the flipper. As the appendage is used for flying and propelling underwater, it is less efficient than a wing at flying, and less efficient than a flipper at swimming. An example of these birds is the thick-billed murre or Brünnich's Guillemot. The thick billed murre possesses wings adapted to diving and it is far better at swimming than flying. When it flies, take-off is very awkward for the bird, but once it is airborne it can fly at up to 75 miles per hour, locating fish, squids and crustaceans in bodies of water. Once it has located a prey item, the murre can dive at depths of up to 200 meters to catch it. Underwater, the murre propels itself with the same motion of the wings that it would use to fly (“Thick-Billed Murre” | National Geographic, n.d.).

Architecture of Flippers and their Required Function
The evolution of the limbs of aquatic mammals has led to a flipper structure. This structure has changed from terrestrial limbs in many ways: most marine mammals have encased their forelimb into soft tissue, the proportions of the underlying bone structure have changed as most flippers are roughly at the body trunk, there has been a reduction in the amount of muscle, some tendons have changed to ligaments, the restriction of movement in joints has changed , and subcutaneous and interosseous connective tissues as well as blood vessels have changed in such a way that tissue density augmented, and artery and vein evolved to improve heat exchange (Norris, 1966). All these modifications have occurred to fulfill the required function of these limbs.
Various flipper structures from different marine mammals result in distinct functions. The bone structure varies between species as seen in Fig. 3.
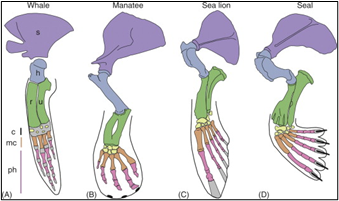
Cetaceans, which are aquatic mammals in the infraorder Cetacea (Editors of Encyclopedia Britannica, “List of cetaceans”), have less muscular and soft tissue structures than other marine mammals' flippers. They are mainly used to stabilize the animal and to help it turn around. The most rapid cetaceans (Fig. 4) have long flippers and thin edges which can generate lift while slow cetaceans (Fig. 5) that need a lot of maneuverability, have broad or spatula-shaped flippers (Sanchez and Berta, 2010).
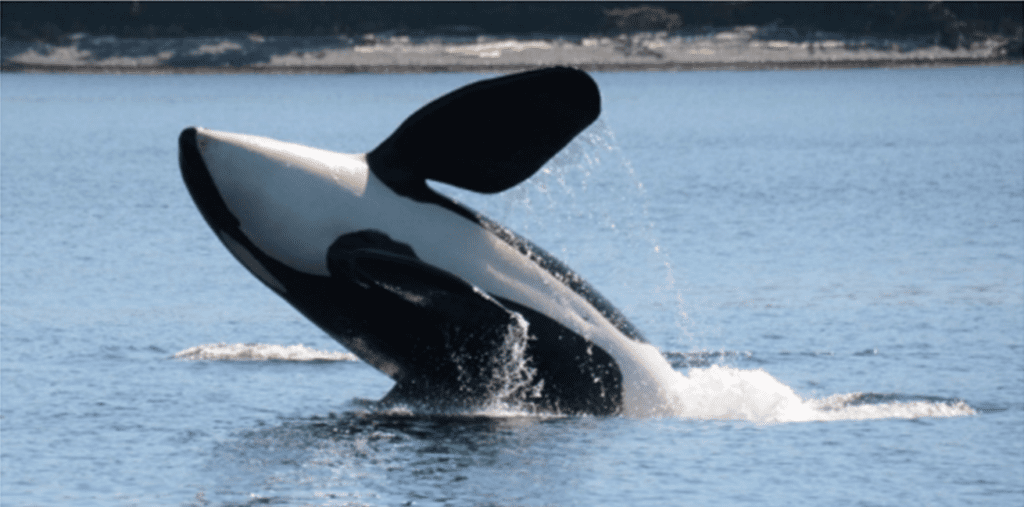
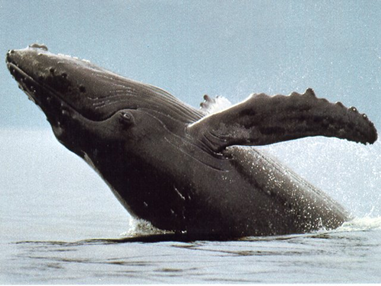
In contrast, sirenians, a group of herbivorous mammals (O'Shea, 2020), use their flippers for orientation and to make little corrections in their trajectory while swimming instead of using them as control surfaces. However, when they are in contact with the seafloor, their flippers are the main propulsion method: they “walk” on the ocean floor, or they paddle. As a result, their flippers, which are held by large, rounded tendons in the proximal and distal limb, are significantly more muscular (Fig. 6).
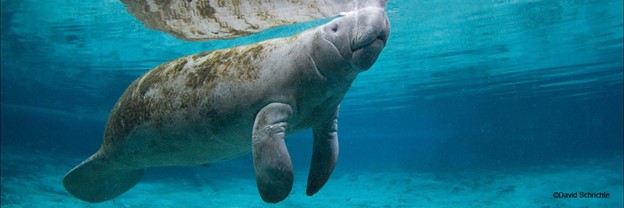
Pinnipeds, also known as seals (Lariviere, 2018), have both forelimb and hindlimb flippers. The fore flippers are used primarily for locomotion in water, but also have limited use on the ground; they put their digits flat and their wrists up, which is not ideal for locomotion on the ground and restricts their displacements. In water, their flippers work similarly to bird wings that flap and give direction. Flippers of Pinnipeds are thin, long, and muscular, with long digits due to the bars of cartilage at their extremity (Fig. 7). Most of their muscles are in the proximal section of the member and can control elbow movements. They also have wrist muscles that are used to generate palmar contractions. These contractions create thrust and give speed to the animal. The polar bears' forelimbs are used as flippers when they swim: they use them as their main source of propulsion. As they need to swim, walk on land or ice, hunt and be able to get out of the water, they have very powerful fore limbs (Fig. 8). However, their limbs resemble more the limbs of terrestrial mammals than flippers (Cooper, 2009).
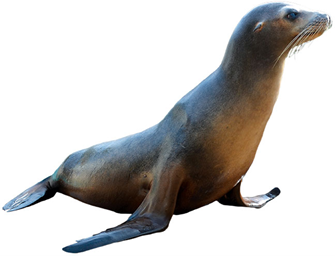
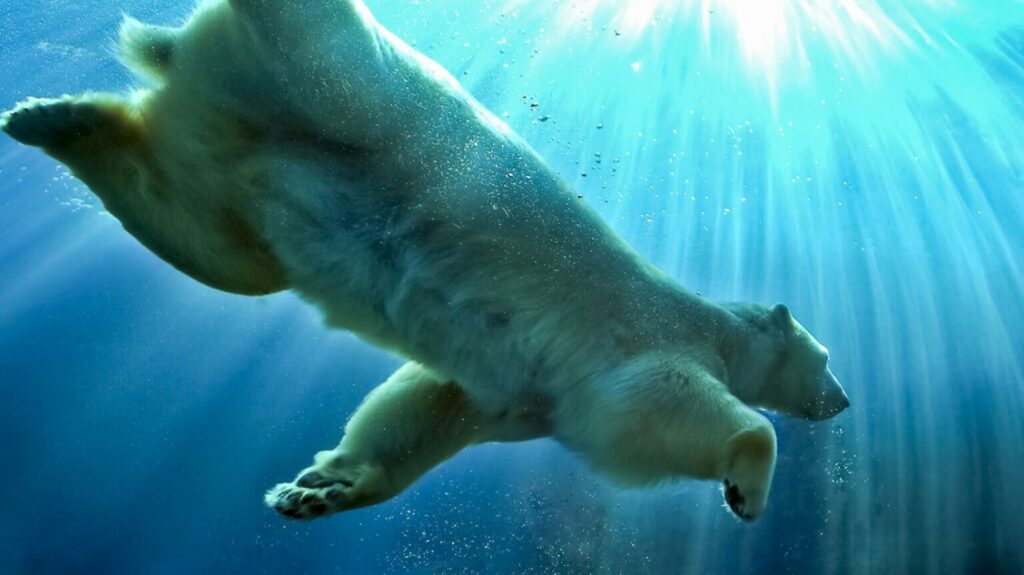
The principal function of flippers is locomotion. They act as hydrofoils and can, in a sense, be compared to a bird's wing (Fish, “Structure and mechanics of nonpiscine control surfaces”). While the main purpose of posterior positioned flukes is to generate thrust, anterior flippers usually generate lift, which is useful for maneuverability and stability (Segre et al., 2016). The movement of flippers includes three distinct types of motion: rolling around the shoulder, forward and backward movements (yaw), and rotation around its own axis (pitch) (“How Plesiosaurs Ruled the Ocean using their Flippers” | Tom Rocks Maths | YouTube, 2019).
The fact that most flippers are flat contributes to decreasing water resistance and helps provide stability (Del Castillo et al., 2014). Therefore, from a hydrodynamic perspective, their shape is critical as it decreases drag and the forces in roll, yaw and pitch directions (Sanchez and Berta, 2010). The leading edge of the flipper is held in place by bones making it strong enough to resist the hydrodynamic forces that it is subject to. To have hydrofoil behavior, flippers are required to be long, rigid and webbed, as this contributes to the increase in the flow manipulation area (Cooper et al., 2008). Penguins' flippers have the same bone structure as birds' wings except that they are shorter and flat. They also have very short feathers which help to minimize friction and turbulence. This allows the penguins to propel themselves through water at very high speeds (Gill and Prevost, 2019). Humpback whales have big bumps, called tubercles (Fig. 9), on the leading edge of their flippers. These tubercles increase their aerodynamicity; this phenomenon is known as the tubercle effect. It works by dividing the water flow into many narrow streams, which results in creating higher flow velocity. It also reduces the flow over the tip of the flipper which results in less parasitic drag. Tubercules can augment lift by about 4.8 % and diminish drag by up to 40 % (Ng et al., 2016). In brief, the shape and the structure of the flipper greatly influences the speed performances and propulsive efficiency of the different animals.
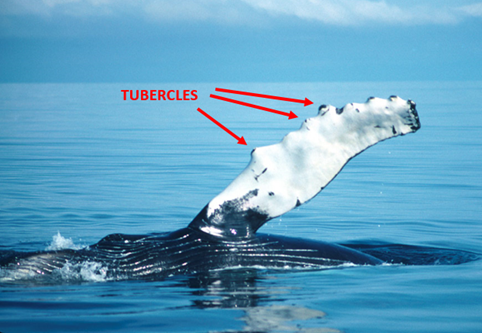
Since most ocean mammals live in very cold and extreme conditions where the water can reach temperatures of approximately -1.7 ℃, they must be able to endure them (Sanchez and Berta, 2010). The pelage of these animals has a significant role in thermoregulation. In penguins, the flippers are covered by a dense layer of feathers that allow them to conserve their heat (Gill et al., 2019). Marine mammals' trunks are enveloped in a layer of blubber for insulation (Kvadsheim and Folkow, 1997). Since flippers are separated from the main body, they are less insulated. Fortunately, being poorly insulated allows them to absorb heat coming from the sun. Blood vessels are also vital when it comes to thermoregulation. Some marine mammals, including seals (“Seal Temperature Regulation” | Dive Blog | Abyss Scuba Diving, 2019) and sea lions, have peripheral blood vessels that allow them to circulate that warm blood to the rest of the body through arteries. Veins surround the arteries and help insulate the warm blood that is brought to the rest of the organism. This process results in improved thermoregulation (“Thermoregulation” | Aquarium Blog | Aquarium of the Pacific, n.d.). In a similar way, dolphins are warm-blooded and must maintain a body temperature between 97 ℃ and 99 ℃, so the dorsal fin serves an additional purpose of acting as a thermoregulator (“Dolphin Fin Structure and Function” | Understand Dolphins, n.d.). The blood vessels of dolphins are located close to the surface in the dorsal fin, and in the case of the body overheating, the fin releases excess body heat into the environment to regulate the dolphin's body temperature (“Dolphin Fin Structure and Function” | Understand Dolphins, n.d.).
Flippers are also used in the alimentation process. Baleen whales often rotate on their own axis (roll), for numerous reasons, including foraging, feeding, and removing their parasites. Although the reason why whales roll during the feeding process is unknown, it is presumed that it increases the prey capture efficiency. These rotations are primarily controlled by the flippers due to their rolling performance (Segre et al., 2016). Also, during foraging, whales use the flat side of their flippers to push the school of fish and facilitate their capture (“Humpback whales use their flippers to help catch fish” | Science News | YouTube, 2019). Sea turtles also use their front flippers to hold on, manipulate and unearth their prey (“What do sea turtles use their flippers for?” | Olive Ridley Project, n.d.). Flippers have other particular uses. For instance, male sea turtles use them during reproduction to grab onto the carapace of the female: the big claw on the end of each flipper allows them to grasp the carapace more easily (“What do sea turtles use their flippers for?” | Olive Ridley Project, n.d.) (Fig. 10).
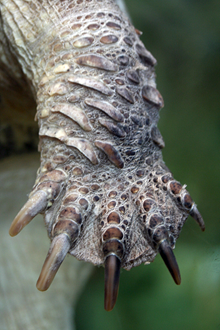
Operation of Flippers and their Required Function
Over hundreds of years of evolution, animals have maximized their bodies to suit their lifestyle. Depending on their environment, habitat, prey, and predators, every animal has adapted to survive. Most importantly, the way they use their bodies increases their efficiency while using minimal energy. Flippers are significant contributors to this. These are an adaptation of a flat limb, allowing a greater surface area to push more water, facilitating movement (Mueller, n.d.). They are found in animals across various vertebrate groups, including mammals, fish, and birds. Almost universal among all mammals and birds, is the ability to swim. They are secondary swimmers, meaning they have descended from terrestrial ancestors who may have returned to water for access to food or to escape from terrestrial predators. On the contrary, the entire evolutionary history of fish is aquatic, making them primary swimmers (Wada, 2019). Moreover, the use of flippers by seals, fins by sharks, and webbed feet by ducks is observed. Despite their similarities in easing the animal's navigation through water, each one works uniquely, specific to its function.
Pinnipeds
Alive today, there are thirty-three species of pinnipeds. The three main groups include the walrus, the eared seal, and the earless seals. Despite their name, earless seals, also known as phocid seals, have ears hidden beneath the surface of their skin. The animals typically live in cold-water environments. This caused the development of thick layers of fat and dense fur (“Seals” | National Geographic, n.d.). In addition, their pectoral and hind limbs have evolved into flippers. This transformation caused them to lose strength as it is difficult for some seals to support their raised body weight while out of the water (Wada, 2019). As they are semi-aquatic mammals, seals spend periods of their life on land or sea ice, typically during mating and birthing seasons (“Seals” | National Geographic, n.d.). However, while in the water, these flippers allow them to swim far distances very quickly. Unlike other mammals that generate thrust with the back ends of their body, using their tail to propel through the water, phocid seals take advantage of their fore-flippers. They use a particular motion named a “clap”, where they generate a forward propulsion in a wide sweeping motion. Data from tracking these seals reveals that their flippers twist as the clap happens. The water is cupped in front of their body and then pushed backwards, shooting the seal forward accordingly. Just one strong clap is enough to generate the thrust necessary to support the seal gliding through the water. Following this, seals tuck their flippers against their bodies, forming an aerodynamic torpedo shape. Consequently, they are free to twist and roll with little additional energy (Morsink, 2017).
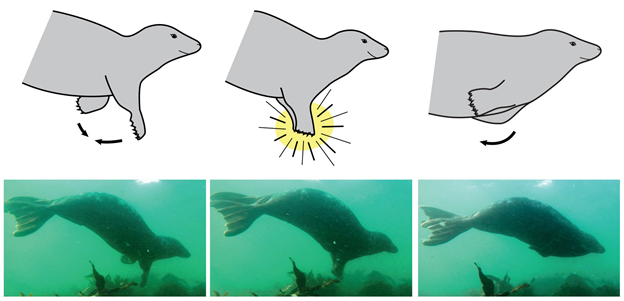
Moreover, a study was conducted where individual seals were filmed swimming in a flume, a man-made channel with a water current created by two hydraulic turbines. By replicating their natural environment, the researchers were able to witness how powerful their flippers are (Fish et al., “Kinematics and estimated thrust production of swimming harp and ringed seals”). Along with the motion of their fore-flippers, these seals incorporated lateral flexions of their axial body and alternate lateral sweeps of their hind flippers. Together, locomotion was accomplished, allowing them to reach velocities of 1.42 m/s. As they swam faster, the frequency of this propulsive cycle increased (Fish et al., “Kinematics and estimated thrust production of swimming harp and ringed seals”). At the same time, the maximum angle of attack of the flipper, which is between the tangent of the flipper's path and the axis of the hind flipper, decreased. All these observations support the claim that as the flippers act as hydrofoils, the efficiency of the seal is thus increased (Fish et al., “Kinematics and estimated thrust production of swimming harp and ringed seals”). This is further proven by the high propulsive efficiency, calculated at approximately 85 %. This number proves that almost all the energy exerted is converted into kinetic energy (Fish et al., “Kinematics and estimated thrust production of swimming harp and ringed seals”). Altogether, using both their fore and hind flippers, seals may manipulate the water around them to propel themselves forward. This allows them to swim speedily, and even generate enough thrust to lift their body out of the water.
Sharks
All four hundred species of sharks alive today spend their entire life in the water. The smallest of them is the dwarf lantern shark that only grows seven inches long, while the largest of them is the whale shark that can measure up to 60 feet. Although very few species actually lie near the top of the food chain, they all have relatively the same features that enable them to be the vicious predators that they are (Leurs, 2017). Their body structure resembles an airplane. As the tail swings back and forth, it acts as a propeller, moving their body forward. This motion sends water around its fins, creating lift (Harris, 2001). This is controlled by changing the angles of the pectoral and pelvic fins. These are wide flat surfaces that are supported by internal rays named ceratotrichia, and appear in pairs, one on each side of the body (“Shark Anatomy” | Shark Trust, n.d.). By modifying their angle, a new path for the water circulating around them is determined. As the fins tilt up, pressure rises below the fin, creating an upward lift. Contrarily, as the fins tilt down, pressure drops below the fin, pushing the shark downwards. Furthermore, they have a dorsal fin on top of their body and an anal fin on the bottom.
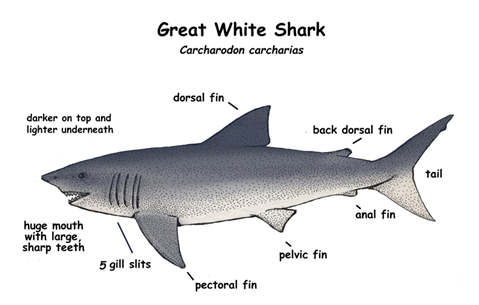
It is commonly believed that the dorsal fin simply provides balance for the shark, however, a recent study proves that it is not limited to a stabilizing role. Two species, spiny dogfish and bamboo sharks, were observed through a series of consecutive images. From these, the stroke-average longitudinal and lateral velocity components of the sharks were calculated.
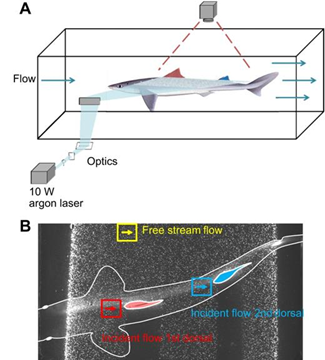
In bamboo sharks, the path behind the front and back dorsal fins, showed increased net flow velocity, revealing a thrust contribution while swimming. Conversely, in spiny dogfish, a velocity deficit in the path behind by the first dorsal fin yet an accelerated flow being the second dorsal fin, appeared. This indicates that the first fin experiences net drag, while the second fin aids in propulsion. These results show that the function of dorsal fins can differ among species (Maia et al., 2017). As all fins arrange themselves, the shark experiences efficient maneuverability, allowing it to cruise at high speeds, stop suddenly, and control sharp turns in every direction. This is significant as they become effective hunters, moving quicker and with more control than any of their prey (Harris, 2001).
Webbed Feet
The ability to fly, walk, and swim, is mastered by 400 species of birds with webbed feet, alive today (Sundstrom, 2020). Webbed feet are a specialized limb found in many semi-aquatic mammals. These are comparable to flippers as they are an adaptation of flat surfaces that ease paddling through water. Ducks can swim at the surface of the water or under with the help of a thin interdigital layer between its toes. As it pulls its foot backwards, its toes separate, causing the web to spread out. This exposes a large surface area that can push a great volume of water, propelling the bird through the water. Then, on the forward stroke, the duck pulls its toes together, folding up the web. By reducing its drag, the foot becomes less resistant, preventing the duck from moving backwards (“Adaptations- Feet: Swimming” | Project BEAK, n.d.). Simultaneously, the duck can raise its foot away from its body, giving itself extra lift and speed. When the angle of attack of the foot and the relative water velocity are considered, a hydrodynamic lift is possible (Sundstrom, 2020). This allows the duck to easily glide through the water and prepare for the following stroke. To continue, there are two main variations of webbed feet: totipalmate and palmate as shown in Fig. 14. Most birds have three toes on the front of their foot and a fourth toe by the back. The term “totipalmate feet” refers to all four toes being united by ample webbing whereas the term “palmate feet” refers to the front three toes joined by webbing without the back toe that does not help push the bird through the water.
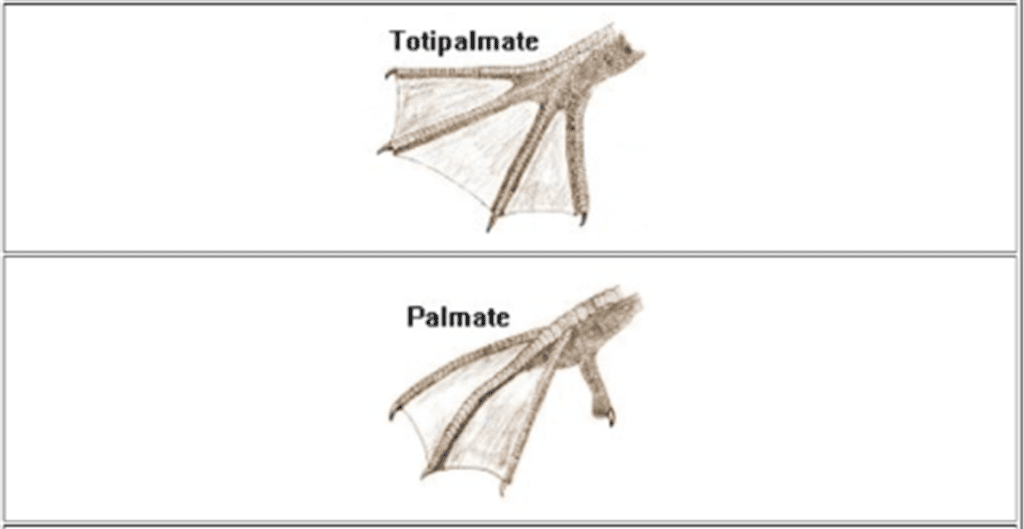
Furthermore, most birds that swim have their legs and feet near the rear of their body. This adaptation is beneficial while propelling through water, but a disadvantage while waddling on land. Due to the imbalance of weight spread, it becomes more difficult for these birds to walk. Despite this, webbed feet are useful while on mud as it prevents birds from sinking (“Adaptations- Feet: Swimming” | Project BEAK, n.d.). Aside from swimming at the surface of the water, ducks spend a lot of time at the bottom of lakes and ponds, feeding. These divers primarily eat plants, insects and little fish (Animals Network Team, 2018). To be able to not only reach the bed, these ducks use their webbed feet to create a propulsive force that moves them underwater. However, to be able to hold their position at the bottom, these ducks must paddle constantly to resist the buoyant force pulling them to the surface. Almost 98 % of this propulsive force in the sagittal plane of the duck, essentially down the middle of its body separating the left and right side, worked to oppose buoyancy (Ribak et al., 2010). Altogether, it is obvious that along with their ability to fly, birds have widely benefited from using their webbed feet in the water.
Comparative Study of Flippers from Different Animals
Flippers are a common structure amongst several vertebrates. Fish, aquatic mammals, and aquatic birds all possess flippers or flipper-like appendages that help them navigate marine environments. Fish are mostly cold-blooded vertebrates with fins (Parenti and Weitzman, 2019), which differ from flippers in that they have no skeletal structure, are predominantly made of cartilage, and did not evolve from limbs (“Fin vs. Flipper” | Key West Aquarium, n.d.). Aquatic mammals live the majority, if not all, of their lives nearby or in aquatic environments, and they rely on the marine ecosystem for survival (“Marine Mammals” | National Oceanic and Atmospheric Administration, n.d.). Marine mammals can have both flippers and fins which serve different purposes. Aquatic birds, like aquatic mammals, spend much of their lives by the water, either floating, swimming, or diving to catch fish (Robb, n.d.). To facilitate their aquatic lifestyle, marine birds have webbed feet (“Adaptations – Feet: Swimming” | Project BEAK, n.d.). Although all these limbs serve similar functions for these aquatic vertebrates, the flipper, fin, and webbed foot are structurally and evolutionarily distinct.
Sharks
At the mention of fins, the vision of a shark fin moving threateningly through the ocean often comes to mind, but the purpose of shark fins and the fins of fish in general goes far beyond inciting fear. Fish have several different forms of fins, and they are amongst the most distinguishing features, making them a useful tool for identifying different species of fish (“Fish Fins: Modifications and Functions” | Biology EduCare, n.d.). Two types of fins that most fish possess are the median fins and the paired fins (“Fish Fins: Modifications and Functions” | Biology EduCare, n.d.) (Fig. 15).
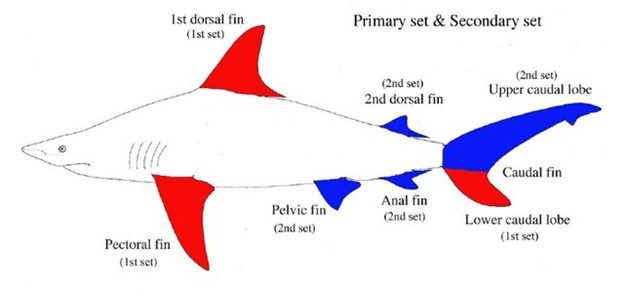
Median fins run along the middle plane of the body and are comprised of the dorsal, anal, and caudal fins while paired fins come in pairs homologous to human limbs and consist of pectoral and pelvic fins (“Fish Fins: Modifications and Functions” | Biology EduCare, n.d.). A shark's fins, which are rigid and elongated (“Sharks” | Sharks Info, n.d.), play a fundamental role in the stabilization, buoyancy, propulsion, and steering of the fish (“Aquatic Species- Sharks: Shark Anatomy” | Fisheries and Oceans Canada | Government of Canada, n.d.). Sharks have either one or two dorsal fins, depending on the species (“Sharks” | Sharks Info, n.d.). The first dorsal fin is located behind the animal's head and is the aforementioned fin that is frequently seen above the water whereas the second dorsal fin, if there is one present, is further down the shark's torso, much smaller and positioned in line with the pelvic fins (“Sharks” | Sharks Info, n.d.). The dorsal fins are mainly stabilizing as they keep the fish upright by preventing tipping and rolling of the animal (“Sharks” | Sharks Info, n.d.). In some species of sharks, the dorsal fins have defensive mechanisms in place, such as sharp spines or the ability to secrete venom (“Aquatic Species- Sharks: Shark Anatomy” | Fisheries and Oceans Canada | Government of Canada, n.d.). Anal fins are located between the pelvic fins and the tail on the ventral side and aid in stability, although they are not present on all species (“Sharks” | Sharks Info, n.d.).The tail, also known as the caudal fin, is most often a vertically expanded fin with an upper lobe and a lower lobe, that vary in shapes and sizes across different species (“Aquatic Species- Sharks: Shark Anatomy” | Fisheries and Oceans Canada | Government of Canada, n.d.). Just ahead of the caudal fin, at its base, is the caudal peduncle (“Fish Fins: Modifications and Functions” | Biology EduCare, n.d.). It functions like the motor of the caudal fin whose primary purpose is to provide thrust and propel the shark forward by moving it from side to side (“Aquatic Species- Sharks: Shark Anatomy” | Fisheries and Oceans Canada | Government of Canada, n.d.). The fin does not allow for reverse movement, so the fish must either drift backwards or turn around to be able to continue in the desired direction (“Sharks” | Sharks Info, n.d.). As the upper lobe of the caudal fin produces more thrust, the pectoral fins of the animal along with the shape of its body provide the necessary lift to counteract the downwards force of the thrust from the upper lobe (“Aquatic Species- Sharks: Shark Anatomy” | Fisheries and Oceans Canada | Government of Canada, n.d.). The pectoral fins are present on both sides of the body, just behind the head of the fish (“Sharks” | Sharks Info, n.d.). Along with providing a lifting force, these fins are also used for steering (“Fish Fins: Modifications and Functions” | Biology EduCare, n.d.). The final type of shark fin is the pair of pelvic fins, located further down the body than the pectoral fins, on the ventral side (“Sharks” | Sharks Info, n.d.). The pelvic fins help stabilize, slow down, and move the fish up and down (“Fish Fins: Modifications and Functions” | Biology EduCare, n.d.). In males, the pelvic fins are modified into claspers, which are copulatory organs all of which contribute to the stable propulsion of fish through the water (“Aquatic Species- Sharks: Shark Anatomy” | Fisheries and Oceans Canada | Government of Canada, n.d.).
That dorsal fin moving ominously through the ocean may not even belong to a shark at all, but rather to an aquatic mammal with a dorsal fin that appears the same from afar but has differences upon closer inspection.
Dolphins
Dolphins and sharks are a fascinating example of convergent evolution, the process by which different organisms independently evolve similar traits as a result of adaptation to like environments, in this case, the ocean (Wood, 2019). Although sharks and dolphins are not related, their similar streamlined body shapes and fins are analogous structures which help them survive in their marine environments (“How do analogies evolve?” | Understanding Evolution, n.d.). Dolphins, like sharks, have a dorsal fin located on the top side of the animal. However, dolphins always only have a singular dorsal fin (Fritscher, n.d.). A dolphin's dorsal fin is made of a fibrous connective tissue and serves a similar function to the dorsal fin of a shark, that is, it mainly acts as a stabilizer and prevents the animal from rolling (“Dolphin Fin Structure and Function” | Understand Dolphins, n.d.). Furthermore, the dorsal fin of a dolphin acts as a thermoregulator (refer to the section Architecture of Flippers and their Required Function of this paper)(“Dolphin Fin Structure and Function” | Understand Dolphins, n.d.). Another notable difference is that the shape of the dorsal fin varies between dolphins and sharks (Fritscher, n.d.). Where the fin of a shark stands straight up from its back, a dolphin's dorsal fin is falcate, or curved backward towards the rear of the dolphin (Fritscher, n.d.) (Fig. 16). This fin has a notched pattern on the trailing edge, unique to each dolphin, much like human fingerprints are distinctive to each person (“Dolphin Fin Structure and Function” | Understand Dolphins, n.d.). Due to this, this pattern can be used to help identify individual dolphins (“Dolphin Fin Structure and Function” | Understand Dolphins, n.d.).
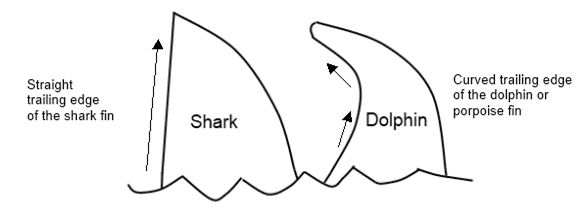
Moreover, dolphins have both fins and flippers, unlike sharks. The front limbs of a dolphin are called the pectoral flippers and have a bone structure similar to that of the human arm, wherein they have a wrist and hand. This strongly indicates the evolution of dolphins from land mammals (“Dolphin Fin Structure and Function” | Understand Dolphins, n.d.). Their flippers, like the pectoral fins of sharks, are used for steering (Fritscher, n.d.). Similar to the dorsal fin, the blood vessels of the pectoral flippers are close to the surface, assisting in thermoregulation by conserving body heat. They can do this by adjusting the circulation of blood to the flippers under varying water temperatures (“Dolphin Fin Structure and Function” | Understand Dolphins, n.d.). To continue, the tail region of a dolphin is made up of the peduncle and the flukes (“Dolphin Fin Structure and Function” | Understand Dolphins, n.d.). The peduncle is the muscular section of the tail located between the dorsal fins and the flukes that generates much of the mammals' power for propulsion, similar to the peduncle of the shark. The peduncle flattens into two wide horizontal lobes known as the flukes, which are responsible for the propulsion of the animal by moving up and down. This varies from the tail of the shark in the direction of movement (“Dolphin Fin Structure and Function” | Understand Dolphins, n.d.). In summary, although the bodies and fins of sharks and dolphins look similar, they differ in structure, function, and evolutionary path.
Penguins
Another instance of convergent evolution is demonstrated by penguins. The penguin is an aquatic bird whose wings have evolved into flippers to facilitate their marine lifestyle, organs analogous to the flippers of dolphins and sharks (Mayntz, 2019) (Fig. 17).
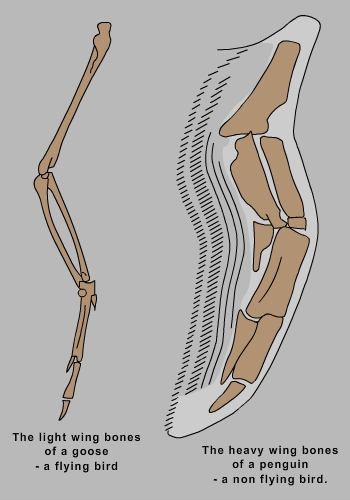
The bones of the wing flattened and broadened and the joint of the elbow and the wrist nearly fused over time, resulting in a flat, thin and tapered flipper with a blunt, round tip (“Flippers” | Pinguins Info, n.d.). Because the penguin's wing appendages were modified into flippers, these birds cannot fly (Mayntz, 2019). The penguin relies on its paddle-like muscular flippers for propulsion through the water and on its feet and tail for steering (Mayntz, 2019). The strong breast musculature of the penguin is responsible for the movement of the flippers (“Flippers” | Pinguins Info, n.d.). The pectoralis major pulls the appendage downwards while the Supracoracoideus, the smaller breast muscle, pulls the flipper upwards, resulting in high-speed propulsion through the ocean (“Flippers” | Pinguins Info, n.d.). Although the motion of the flipper is similar to that of the wings of a bird in flight, there are some key differences (Mayntz, 2019). The flipper of a penguin can only be moved from the shoulder as the elbow and wrist are completely fused, giving the bird more power for propulsion but limiting the flexibility (Mayntz, 2019). The fused elbow and wrist reduce the risk of injury from the high-pressure resistance of water (Mayntz, 2019). The flippers of penguins are covered densely with short, stiff feathers which provide insulation and aid in minimizing drag to allow for faster swimming (Mayntz, 2019). The primary use of the flipper in penguins is for swimming, but they serve other purposes as well. Because the flippers are so muscular and strong, they are an effective weapon of defense against predators (“Flippers” | Pinguins Info, n.d.). In addition, the flippers are a useful tool for communication between penguins, with certain motions conveying different meanings such as anger, dominance, and courtship (Mayntz, 2019). While the flippers are most useful underwater, on land they can be used for balance, propulsion while sliding on ice, and to conserve body heat (Mayntz, 2019). Although penguins are the only birds that have real flippers, an adaptation common amongst other species of aquatic birds is webbed feet (“Adaptations- Feet: Swimming” | Project BEAK, n.d.).The main purpose of webbed feet is the same as that of a flipper: to propel the animal through the water (refer to the section Operation of Flippers and their Required Function of this paper) (“Fish Fins: Modifications and Functions” | Biology EduCare, n.d.). Penguins have both flippers and webbed feet (Mayntz, 2019).
Conclusion
Altogether, this analysis illustrates the complexity of flippers. Regarding their functions, many parameters come under consideration. Their surface area, range of motion, and permeability greatly affect their efficiency as they displace as much water as possible while expending minimal energy. Nevertheless, a surface area too large can be counterproductive. Another important consideration is the number of flippers an animal has. Most aquatic mammals only have two flippers because a greater number would result in a much higher energy cost. In rarer cases, animals such as the thick-billed murre have hybridized their wings and flippers throughout evolution, resulting in the ability to “fly underwater”. From an architectural point of view, the morphology of flippers of various animals is intrinsically linked to their functions. Their shape directly influences hydrodynamics and their composition has a major effect on thermoregulation. Regarding their operation, specific combinations of movements can maximize their underwater propulsion efficiency. Moreover, diverse animals have distinct behaviors, which directly influence their mode of operation. As a result, predators develop enhanced agility and control, giving them a significant advantage over their prey. Some animals use their flippers differently, depending on the surrounding environment. Consequently, although aquatic species have acquired similar adaptations — through convergent evolution — that facilitate their lives in a marine environment and serve the similar purpose of providing balance and propulsion through water, it is clear, upon analyzing the fins of sharks, flippers and fins of dolphins, and flippers and webbed feet of penguins, that there are differences in the shapes, structures, and functions of these limbs.
Appendix
“How Plesiosaurs Ruled the Ocean using their Flippers.”, 22 March 2019, Tom Rocks Maths, YouTube, https://www.youtube.com/watch?v=chfG229nLZI.
“Humpback whales use their flippers to help catch fish.”, 16 October 2019, Science News, https://www.youtube.com/watch?v=9s0NYJrubGY.
References
(NOAA), N. O. a. A. A. (2019, 1 Feb 2019). Marine mammals. Retrieved from https://www.noaa.gov/education/resource-collections/marine-life/marine-mammals
Adams, P. (2020). Polar bears make a comeback despite melting ice caps. Retrieved from https://voltafuturepositive.com/2020/11/12/polar-bears-make-a-comeback-despite-melting-ice-caps/
Amsel, C. (n.d.). Shark (Great White). In. exploringnature.org: Exploring Nature. Retrieved from https://www.exploringnature.org/db/view/Shark-Great-White
Aquarium, K. W. (n.d.). Fin vs. Flipper. Retrieved from https://www.keywestaquarium.com/fin-vs-flipper
Ashley, S. (2004). Bumpy flying. Scalloped flippers of whales could reshape wings. Scientific American, 291(2), 18,-20. Retrieved from http://europepmc.org/abstract/MED/15298109
BEAK, P. (n.d.). Adaptations- Feet: Swimming Retrieved from http://projectbeak.org/adaptations/feet_swimming.htm#:~:text=Ducks%2C%20geese%2C%20and%20swans%20all,spread%2Dout%20toes%20would%20push
Britannica, E. o. E. (2016, 3 Mar 2016). List of cetaceans. Retrieved from https://www.britannica.com/topic/list-of-cetaceans-2057165
Brodie, P. F. (1975). Cetacean Energetics, an Overview of Intraspecific Size Variation. Ecology, 56(1), 152-161. doi:10.2307/1935307
Canada, G. o. (n.d.). Aquatic Species- Sharks: Shark Anatomy. Retrieved from https://www.dfo-mpo.gc.ca/species-especes/sharks/anatomy-eng.html
Club, S. t. M. (n.d.). Sirenians Of The World: Manatees. Retrieved from https://www.savethemanatee.org/manatees/sirenians-of-the-world/
Coast, B. o. t. C. (n.d.). Killer Whale. Retrieved from https://www.centralcoastbiodiversity.org/killer-whale-bullnbsporcinus-orca.html
Cooper, L. N. (2009). Forelimb Anatomy. In W. F. Perrin, B. Würsig, & J. G. M. Thewissen (Eds.), Encyclopedia of Marine Mammals (Second Edition) (pp. 449-452). London: Academic Press.
Cooper, L. N., Sedano, N., Johansson, S., May, B., Brown, J. D., Holliday, C. M., . . . Fish, F. E. (2008). Hydrodynamic performance of the minke whale (Balaenoptera acutorostrata) flipper. Journal of Experimental Biology, 211(Pt 12), 1859-1867. doi:10.1242/jeb.014134
Del Castillo, D. L., Panebianco, M. V., Negri, M. F., & Cappozzo, H. L. (2014). Morphological analysis of the flippers in the Franciscana dolphin, Pontoporia blainvillei, applying X-ray technique. Anat Rec (Hoboken), 297(7), 1181-1188. doi:10.1002/ar.22908
Dictionary, M.-W. (Ed.) (n.d.-a). flipper. In. Merriam-Webster. merriam-webster.com. Retrieved from https://www.merriam-webster.com/dictionary/flipper
Dictionary, M.-W. (Ed.) (n.d.-b). fin. In. Merriam-Webster. merriam-webster.com. Retrieved from https://www.merriam-webster.com/dictionary/fin
Diving, A. S. (2019). Seal Temperature Regulation. Retrieved from https://www.abyss.com.au/en/blog/viewpost/185/seal-temperature-regulation
Dolphins, U. (n.d.). Dolphin Fin Structure and Function. Retrieved from https://understanddolphins.tripod.com/dolphinfinstructurefunction.html
EduCare, B. (n.d.). Fish Fins: Types, Modification and Functions. Retrieved from https://biologyeducare.com/fish-fins-its-types-and-functions/
EnergiMedia. (2018). Humpback whale flippers inspire Canadian design of highly efficient wind turbine blade. In. energi.media. Retrieved from https://energi.media/innovation/canadian-inventors-turbine-humpback-whales-increasing-wind-efficiency/
Evolution, U. (n.d.). How do analogies evolve? Retrieved from https://evolution.berkeley.edu/evolibrary/article/similarity_ms_08
faolruadh. (2011). Snapping Turtle Claw. In (Vol. 600x900px 250.94 KB). deviantart.com. Retrieved from https://www.deviantart.com/faolruadh/art/Snapping-Turtle-Claw-201641675
Fish, F. (2009). Biomimetics: Determining engineering opportunities from nature. Proceedings of SPIE – The International Society for Optical Engineering, 7401. doi:10.1117/12.824106
Fish, F. E. (2004). Structure and mechanics of nonpiscine control surfaces. IEEE Journal of Oceanic Engineering, 29(3), 605-621. doi:10.1109/JOE.2004.833213
Fish, F. E., Innes, S., & Ronald, K. (1988). Kinematics and estimated thrust production of swimming harp and ringed seals. Journal of Experimental Biology, 137, 157-173.
Fish, F. E., Weber, P. W., Murray, M. M., & Howle, L. E. (2011). The tubercles on humpback whales' flippers: application of bio-inspired technology. Integr Comp Biol, 51(1), 203-213. doi:10.1093/icb/icr016
Fritscher, L. (n.d.). How to Tell the Difference between Shark and Dolphin Fins. In. animals.mom.com. Retrieved from https://animals.mom.com/tell-difference-between-shark-dolphin-fins-7161.html
Fun, O. o. (n.d.). The Difference: Seal vs. Sea Lions Retrieved from https://www.oceansoffun.org/the_difference
Geographic, N. (n.d.-a). Seals. Retrieved from https://www.nationalgeographic.com/animals/mammals/facts/seals-pinnipeds-walruses-sea-lions
Geographic, N. (n.d.-b). Thick-Billed Murre. Retrieved from https://www.nationalgeographic.com/animals/birds/facts/thick-billed-murre
Gill, F., & Prevost, J. (2019). penguin. Retrieved from https://www.britannica.com/animal/penguin
Handwerk, B. (2013). Why Did Penguins Stop Flying? The Answer Is Evolutionary. Retrieved from https://www.nationalgeographic.com/travel/article/131320-penguin-evolution-science-flight-diving-swimming-wings
Harris, T. (2001). How Sharks Work. Retrieved from https://animals.howstuffworks.com/fish/sharks/shark2.htm
Hocking, D., Burville, B., & Marx, F. G. (2020). Amazing New Footage Reveals Grey Seals Clapping Underwater to Communicate. Retrieved from https://www.sciencealert.com/scientists-caught-seals-clap-talking-to-each-other-under-the-water-on-film
Info, P. (n.d.). Flippers. Retrieved from https://www.pinguins.info/Engels/Lichaam_eng.html
Kvadsheim, P. H., & Folkow, L. P. (1997). Blubber and flipper heat transfer in harp seals. Acta Physiologica Scandinavica, 161(3), 385-395. doi:10.1046/j.1365-201X.1997.00235.x
Lariviere, S. (2018, 11 Oct 2018). Pinniped. Retrieved from https://www.britannica.com/animal/pinniped
Leurs, G. (2017). Are sharks at the top of the food chain? Retrieved from http://saveoursharks.nl/en/blog-are-sharks-at-the-top-of-the-food-chain/
Long, J. H., Jr., Schumacher, J., Livingston, N., & Kemp, M. (2006). Four flippers or two? Tetrapodal swimming with an aquatic robot. Bioinspir Biomim, 1(1), 20-29. doi:10.1088/1748-3182/1/1/003
Maia, A., Lauder, G. V., & Wilga, C. D. (2017). Hydrodynamic function of dorsal fins in spiny dogfish and bamboo sharks during steady swimming. Journal of Experimental Biology, 220(21), 3967-3975. doi:10.1242/jeb.152215
Mayntz, M. (2019). A Penguin Does Have Wings, But They Are Called Flippers. In. thespruce.com. Retrieved from https://www.thespruce.com/flipper-definition-penguin-wings-385251
Morsink, K. (2017). How Do Sea Lions Swim, Glide and Sometimes Even Nab Humans? Retrieved from https://www.smithsonianmag.com/science-nature/how-do-sea-lions-swim-180963847/
Mueller, J. (n.d.). The Comparison of a Whale and a Dolphin. In. animals.mom.com. Retrieved from https://animals.mom.com/comparison-whale-dolphin-5526.html
Ng, B. F., New, T. H., & Palacios, R. (2016). Effects of leading-edge tubercles on wing flutter speeds. Bioinspir Biomim, 11(3), 036003. doi:10.1088/1748-3190/11/3/036003
Norris, K. S. (1966). Whales, dolphins, and porpoises. Berkeley: Univ. of California Press.
O'Shea, T. (2020, 28 May 2020). Sirenian. Retrieved from https://www.britannica.com/animal/sirenian
Pacific, A. o. t. (n.d.). Thermoregulation. Retrieved from https://www.aquariumofpacific.org/blogs/comments/thermoregulation
Parenti, L. R., & Weitzman, S. H. (2019, 4 Jun 2021). fish. Retrieved from https://www.britannica.com/animal/fish
Project, O. R. (n.d.). What do sea turtles use their flippers for? In. oliveridleyproject.org. Retrieved from https://oliveridleyproject.org/ufaqs/what-do-sea-turtles-use-their-flippers-for
Ribak, G., Swallow, J. G., & Jones, D. R. (2010). Drag-based ‘hovering' in ducks: the hydrodynamics and energetic cost of bottom feeding. PloS One, 5(9), e12565. doi:10.1371/journal.pone.0012565
Ritchison, G. (n.d.). Bird Feet. Retrieved from http://people.eku.edu/ritchisong/birdfeet.html
Robb, A. (n.d.). Aquatic Birds: Types & Examples (Lesson ). Retrieved from https://study.com/academy/lesson/aquatic-birds-types-examples.html. https://study.com/academy/lesson/aquatic-birds-types-examples.html
Robinson, M. (2008). Webbed Feet In (pp. A Mute Swan's webbed feet as seen when the bird is standing on land in Somerset, England). Flickr. Retrieved from https://www.flickr.com/photos/66176388@N00/2312270165/in/photolist-4wjZmZ-bjpcTt-4vDAqk
Safran, R. J., & Nosil, P. (2012). Speciation: The Origin of New Species. Nature Education Knowledge, 3(10). Retrieved from https://www.nature.com/scitable/knowledge/library/speciation-the-origin-of-new-species-26230527/
Sanchez, J. A., & Berta, A. (2010). Comparative anatomy and evolution of the odontocete forelimb. Marine Mammal Science, 26(1), 140-160. doi:https://doi.org/10.1111/j.1748-7692.2009.00311.x
Segre, P. S., Cade, D. E., Fish, F. E., Potvin, J., Allen, A. N., Calambokidis, J., . . . Goldbogen, J. A. (2016). Hydrodynamic properties of fin whale flippers predict maximum rolling performance. Journal of Experimental Biology, 219(21), 3315-3320. doi:10.1242/jeb.137091
Séret, B., Blaison, A., Dagorn, L., & Filmalter, J. (2012). Fin to carcass weight ratio for the silky shark Carcharhinus falciformis in the western Indian Ocean.
SharksInfo. (n.d.). Sharks. Retrieved from https://sharksinfo.com/fins.html/
Silber, E. (2016). These Eleven Wildly Talented Birds Deserve Their Own Gold Medals. Retrieved from https://www.audubon.org/news/these-eleven-wildly-talented-birds-deserve-their-own-gold-medals
Sundstrom, B. (2020, 9 Mar). Webbed Feet Are an Evolutionary Hit. M. Stein (Narrator) J. Kessler (Producer). In. audubon.org.
SurfLook. (n.d.). How to tell if a fin above the water belongs to a shark or dolphin. In. surflook.com. Retrieved from http://www.surflook.com/how-to-tell-if-a-fin-above-the-water-belongs-to-a-shark-or-dolphin/#.X719WxNKhQI
Team, A. N. (2018). Duck. Retrieved from https://animals.net/duck/
Tennett, K. A., Costa, D. P., Nicastro, A. J., & Fish, F. E. (2018). Terrestrial locomotion of the northern elephant seal (Mirounga angustirostris): limitation of large aquatically adapted seals on land? Journal of Experimental Biology, 221(Pt 18). doi:10.1242/jeb.180117
Trust, S. (n.d.). Shark Anatomy. Retrieved from https://www.sharktrust.org/shark-anatomy
Wada, D. (2019). Swimming&Diving. Retrieved from http://mammals-locomotion.com/swimming.html
Wood, C. (2019). What Is Convergent Evolution? In. livescience.com. Retrieved from https://www.livescience.com/convergent-evolution.html