The Design of the Spine
Ahmed Bawany, Bianca Dubois, Yzza M’sahi, Michael Parsons
Abstract
This paper discusses the design of the spine in bison and how these animals support their heads without tiring quickly. Furthermore, the purposes of spinal modeling are discussed along with their real-life applications.
Introduction
The spine is crucial to the function of all vertebrates as it is allows movement, and stabilizes and supports the body. The way the spine achieves this is through a system of nerves, bones, and muscles/ligaments (Panjabi, 1992). The architecture of the spine consists of the spinal cord which acts as a highway for signals to be sent from the brain to the rest of the body through a cord of nerves, thus allowing the body to function. The spinal cord is then protected by the spinal column and runs through a hollow center of each vertebrae making up the spinal column. In addition to protecting the spinal cord, the spine also supports the body by distributing loads whether the vertebrate is bipedal or quadrupedal (Panjabi, 1992). The muscles and ligaments surrounding the spine stabilize the body by restricting excessive spinal movement, thus stopping the separating of spinal bodies.
The need of support for the spine is perfectly displayed by the quadruped the bison. The latter is a large quadruped mammal. The bison is the largest mammal in the North America and, when fully grown, it can weigh up to 2 000 pounds (Millburn, n.d.). This massive load provides an engineering feat for the spinal cord to support the weight of body. The bison also has a substantial-sized head with heavy horns, thus posing the question of how the head itself is supported. The answer is of course a well-designed spine. To support their head, bison developed a hump that is found at the base of their neck. This hump is made up of muscles and is supported by vertebrae with large spinal processes (Millburn, n.d.).This topic will be further discussed later in the paper.
Design of a Bison's Spine
The bison spine is a wonderful example of the versatility of nature. A bison's head is huge and weighs a great deal, yet the bison can support its weight without incurring fatigue. To avoid this, the animal evolved to distribute the weight of its head effectively by developing a hump in its neck. This hump's main purpose is to help support the weight of its head (Cornwall, 2016). This is essential as its skull alone can weigh up to 6 kg (Roskosz and Empel, 1961). While this might not seem much, when considering all the other parts that make up the bison's head, the latter can weigh up as much as 35 kg, a significant amount of weight to support (Kohler, 2012).
The hump, as seen in Fig. 1 and 2, is above the upper thoracic region of the bison's spine and comprises the trapezius muscle (Cornwall, 2016). It is strategically located to mitigate the strain caused by the large head as much as possible. This is accomplished primarily due to the greater spinous process of the bison. The spinous processes serve as the foundation for the muscles required to keep the head properly supported.
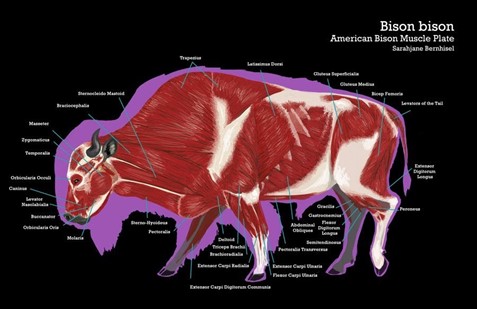
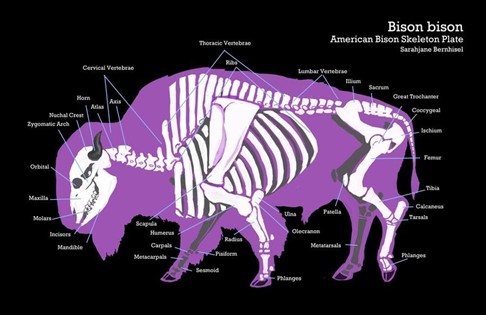
Bison are not the only mammals to have this hump to support their weight, but they do have the greatest spinous process when compared to other animals with humps, such as cattle and bears. However, this is not directly tied to the weight of the head, as might be expected. There are certain animals, such as the Watusi cattle, whose head can weigh double that of the bison, especially in the case of females (Roskosz and Empel, 1961). The largest spinous process can be around 50 cm (20”), as seen in Fig. 3. They need to be this long because the bison needs to have a lot of muscle for the simple task of keeping its head up. However, as previously stated, bison do not have the biggest heads, so why do they have the largest spinous process?
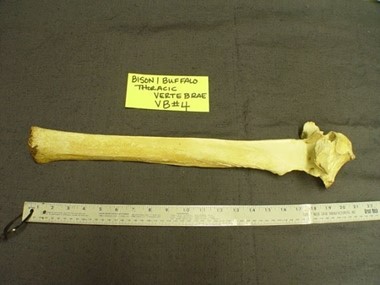
This phenomenon is explained by the eating habits of the different animals. For instance, Watusi cattle are raised in a warmer climate than that of bison. Therefore, their respective eating habits are different, which is what very likely caused this difference in spinous process. Bison eat primarily grass, but also bushes and branches if they are low enough. Due to this, during the winter when the ground is covered in snow, the bison must be able to move the snow to get to their food (Cornwall, 2016). As a result, the bison needs the extra muscle to be able to move snow, which when accumulated, can be extremely heavy. In essence, their head acts as a snowplough. This means that their heads will need to be kept lower to the ground then other animals such as the Watusi cattle.
Another key adaptation made by bison, and other animals in the ungulate category, to help support the weight of their head is the development of the nuchal ligament, also referred to as the paddywack. The nuchal ligament is a strong elastic ligament that connects at various points along the upper vertebrae to the neck vertebrae and to the skull, as seen in Fig. 4 (“Why do bulls have a hump?” | Biology | Stack Exchange, 2016). Humans and other great apes have one, as well as the diplodocids, which were members of the sauropod dinosaurs. Out of these two examples, the bison are like the diplodocids in the way that they used the nuchal ligament to support the weight of their heads (“Why do bulls have a hump?” | Biology | Stack Exchange, 2016; Woodruff, 2017). In essence, the nuchal ligament acts according to the prestress principle. The main aspect of prestress principle is “to counteract the stresses that will result from applied load” (“prestress” | Merriam-Webster, n.d.). The principle is mainly used for concrete, but it also applies to the spine and the nuchal ligaments. The nuchal ligament is what counteracts the tension bearing beam, which is the spine, and the loading stress that is being generated is the weight of the head. Essentially, what is happening is that the nuchal ligament is already stretched when at rest. When the head is extended for various tasks, such as eating, it can easily regain the at-rest state due to the ligament, as shown in Fig. 5, which is an example of how the nuchal ligament works for an ungulate (“Why do bulls have a hump?” | Biology | Stack Exchange, 2016).
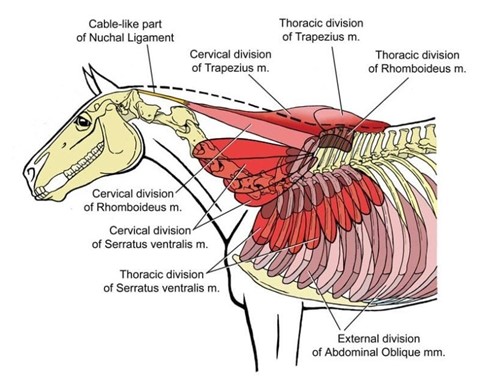
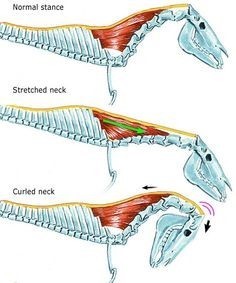
Overall, the main way that bison can support their heads without incurring significant fatigue is due to the hump they have on their back, which, through large spinous process, supports the large amount of muscle required.
The Development of Spinal Modeling
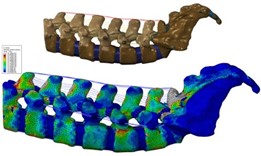
Spinal modelling is crucial in study of spines since the spine and the spinal cord are very delicate and often must remain intact during the study that is being done. The testing methods often bring up ethical dilemmas and they are most often not fully accurate [computational modeling in spine research]. Therefore, computer models have become synonymous with spinal research as they can simulate many scenarios without the use of animals or humans. The improvements in modeling and computational simulations have greatly affected medical and animal research related to the spine (Agarwal et al., 2017). These models are made using CT scanning to and MRI data to make said model as accurate as possible (Megeswaran and Benzel, 2014). Using this method engineers can calculate the locations of stress on the spine, visualize the spine itself, and virtually experiment on the spine under different circumstances that may not be ethically viable in real life (Megeswaran and Benzel, 2014).
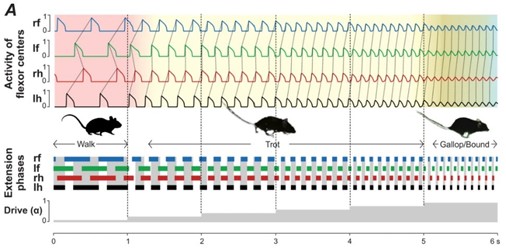
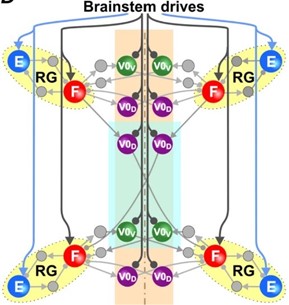
An example of the success in computational spinal modeling is Dr. Simon Danner et al.'s study (2017) on how the brain signals and coordinates the limbs of quadrupeds. To create this model, sensors were put on a rat while it was walking, trotting, and galloping. From the data acquired, Danner's team was able to extrapolate this data to create a computational circuit that showed the pathway of the signals from the brainstem to the rest of the body. The use of a computational model allowed them to ignore other factors and focus specifically on the interest of their study which was the role of the central nervous system in the interactions of rhythms generation circuits and their regulation from the brainstem (Danner et al., 2017).
Trying to Cure Spinal Injuries Using Animals' Model
Walking again after a spine injury has long been considered impossible for humans. The spine protects the spinal cord which establishes a link between the brain and the muscles thus transmitting information. Hence, the spinal cord allows our muscles and our whole body to move. An accident may damage the spine, compromising the functioning of the whole body and sometimes causing a paralysis. Indeed, the spinal cord is now unable to correctly transmit and receive information to and from our muscles and the messages from the brain cannot reach beyond the nervous system. To maintain this transfer of information, scientists and doctors created systems based on animal models (Nathan, 2016).
While trying to find solutions, doctors often base themselves on animal model. When an injury affects the upper spine, the communication between the brain and the lower spinal cord may be interrupted. Even though the motor cortex and spinal neurons remain partially functional, they are unable to coordinate their activity. Thus, neuroscientists have created a wireless “brain-column interface” (Fig. 9) that aims to bypass spinal cord damage. Indeed, this interface acts as a bridge between the brain and spine and thus restores the walking movement on a leg which is temporarily paralyzed (News Staff, 2016).
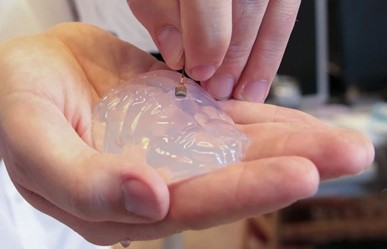
A microelectrode (Fig. 9) was implanted in the part of the brain of the monkeys which intend to control movements. The brain implant reads electrical activity peaks corresponding to instructions for moving the legs and is intended to send those spikes to a nearby computer. The implanted chip aims to support the role of the spinal cord; therefore, the peaks of activity are analyzed, and instructions are sent to another implant in the spine to electrically stimulate the appropriate nerves (Capogrosso et al., 2016, as cited in Gallagher, 2016).
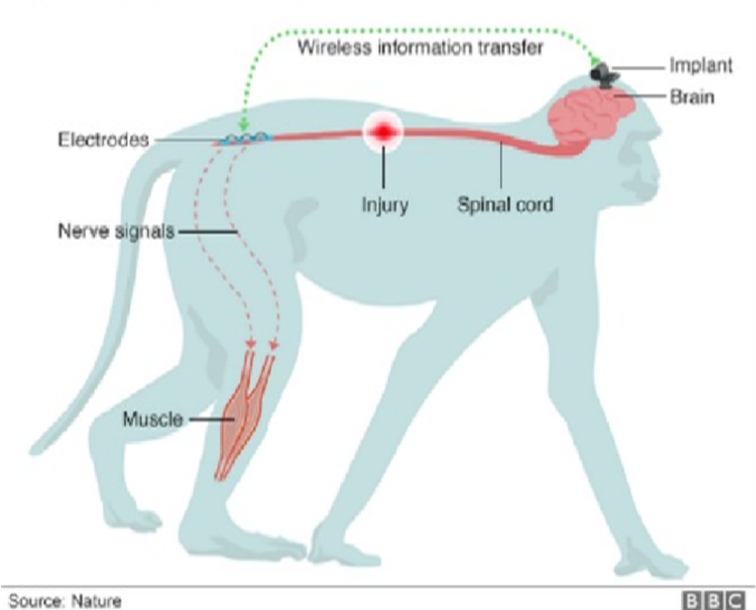
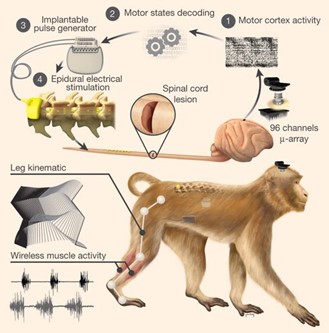
The results of these experiments were conclusive for most of the monkeys tested. Indeed, one of the monkeys regained the use of its legs and was able to walk again (Fig. 12). Thanks to this chip implanted in the brain and connected to the spine, scientists have succeeded in simulating the functioning of the spinal cord and managed to cure the paralysis of the treated macaque (Capogrosso et al., 2016).
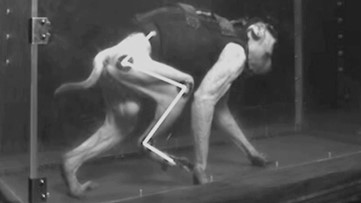
Even though those experiences have been conducted on animals so far, the promising results leave hope for curing human suffering from paralysis in the future.
Conclusion
The spine is crucial in all vertebrates as it protects the spinal cord, allows movement, stabilizes, and creates structure in the body. The bison demonstrates how the architecture of the spine itself can aid the animal in a more specific way. This is proven by the special design of the thoracic vertebrae at the base of its neck to help stop muscle fatigue due to keeping its head up. With the large spinous process on their thoracic vertebrae, bison can support the mass of muscle that creates the hump on their back.
The computational modeling of the spine has been a major advancement in science and engineering as it provides a safe and ethical way to experiment on the spines of animals without injuring them. Engineers and scientists use a variety of devices to create these models such as CT and MRI scanning. Once a 3D model is made, the researcher can choose what stressors to place on the model and use the data to substantiate their claims. Another form of spinal modeling is the use of animals in the place of humans in spinal research. This type of modeling is used often for spinal injuries and uses monkeys as the model.
The improvement of models may one day cut out physical experiments on the spine, thus stopping the ethical dilemmas faced with spinal research. There is a long way to go until this point however, promising advancements are always being made in simulations and modeling.
References
Agarwal, G., Robertson, M. A., Sonar, H., & Paik, J. (2017). Design and Computational Modeling of a Modular, Compliant Robotic Assembly for Human Lumbar Unit and Spinal Cord Assistance. Scientific Reports, 7(1), 14391. doi:10.1038/s41598-017-14220-3
Bennet, D. (2020). Equine Reciprocating Systems: Examining the Shoulder to Thorax Junction. Retrieved from https://www.americanfarriers.com/articles/11488-equine-reciprocating-systems-examining-the-shoulder-to-thorax-junction?v=preview
Capogrosso, M., Milekovic, T., Borton, D., Wagner, F., Moraud, E. M., Mignardot, J.-B., . . . Courtine, G. (2016). A brain–spine interface alleviating gait deficits after spinal cord injury in primates. Nature, 539(7628), 284-288. doi:10.1038/nature20118
Cornwall, T. (2016). Humps: Bison, Bears and Fergie? In. quailandaardvark.wordpress.com. Retrieved from https://quailandaardvark.wordpress.com/2016/11/08/humps-bison-bears-and-fergie/
Danner, S. M., Shevtsova, N. A., Frigon, A., & Rybak, I. A. (2017). Computational modeling of spinal circuits controlling limb coordination and gaits in quadrupeds. Elife, 6, e31050. doi:10.7554/eLife.31050
Dictionary, M.-W. (Ed.) (n.d.). prestress. In. merriam-webster.com: Merriam-Webster. Retrieved from https://www.merriam-webster.com/dictionary/prestress
Gallagher, J. (2016). ‘Brain wi-fi' reverses leg paralysis in primate first. In. bbc.com: BBC News. Retrieved from https://www.bbc.com/news/health-37914543
Kohler, J. (2012). 6 Amazing Facts You Never Knew About Bison. Retrieved from https://blog.nwf.org/2012/02/6-amazing-facts-you-never-knew-about-bison/
Mageswaran, P., & Benzel, E. (2014). Computational Modeling in Spine Research: A Path Toward More Perfect Implant Performance. In. consultqd.clevelandclinic.org: Cleveland Clinic.
Millburn, N. (n.d.). What Is Inside an American Bison's Hump? In. animals.mom.com. Retrieved from https://animals.mom.com/inside-american-bisons-hump-7796.html
Nathan, S. (2016). Spinal implant allows paralysed primate to walk. In. theengineer.co.uk. Retrieved from https://www.theengineer.co.uk/spinal-implant-allows-paralysed-primate-to-walk/
Panjabi, M. M. (1992). The stabilizing system of the spine. Part I. Function, dysfunction, adaptation, and enhancement. Journal of Spinal Disorders, 5(4), 383-389; discussion 397. doi:10.1097/00002517-199212000-00001
Roskosz, T., & Empel, W. (1961). The Size of the Head and the Height of the Spinous Processes in the Region of the Withers of the European Bison, Bison Bonasus (Linenaeus 1758). Acta Theriologica, 6(10), 9.
Staff, N. (2016). Neuroscientists Restore Leg Movement in Nonhuman Primates. In. sci-news.com. Retrieved from http://www.sci-news.com/featurednews/leg-movement-nonhuman-primates-04353.html
user, S. E. (2016). Why do bulls have a hump? In. biology.stackexchange.com. Woodruff, D. C. (2017). Nuchal ligament reconstructions in diplodocid sauropods support horizontal neck feeding postures. Historical Biology, 29(3), 308-319. doi:10.1080/08912963.2016.1158257