Analysis of the Mechanics of the Spine
Ahmed Bawany, Bianca Dubois, Michael Parsons, and Yzza M’sahi
Abstract
This report explores the purpose of the spine by discussing its architecture and mechanics and how this helps its overall function. The similarities between brittle star arms and the mammalian spine are also investigated.
Introduction
This research paper discusses mechanisms, functionality, and design of the mammalian spine, or the vertebral column. Furthermore, this paper addresses design commonalities between the mammalian vertebral column and the arm of the brittle star.
In nature, the spine, for the most part, is similar from one mammal to another, with animals from the same order having the most similarities.
Brittle stars are echinoderms, which are closely related to sea stars. They are invertebrates, meaning they do not have spines; however, they have mechanisms that closely relate to the function of one. By looking at these similarities, insight can be gained into the mechanisms of this key body part, which can then be applied to technology in the real world.
Importance of the Spine
The spine is one of the most important structures of many different types of animals; with over 50 000 species having vertebral columns. As such, the spine takes on many different forms; however, it generally maintains the same functionality across all the animals that possess it. That function is to support the physical structure of the body, as well as support the nervous system, and enable movement and sensation.
Function of the Spine
The spine is made up of a sequence of vertebrae each separated by intervertebral discs. Together, these two make up the vertebral column that supports the entire body of vertebrates and allows for movement (Betts et al., “The Vertebral Column”). In addition to being an integral part in the structure and movement of all vertebrates, it also protects the spinal cord which is an essential cable of nerves that carry messages from the brain to the rest of the body.
In vertebrates the spine is important when it comes to locomotion. It may be thought that legs are the most important part of locomotion; however, the spinal mechanism is deeply rooted in the system. For example, the extension of the spine in the form of the tail moves the center of gravity of the body to over the hips thus aiding the animal in balance and helping them thrust into motion (Gracovetsky, 1985). In addition, the length of the spine is the sole method of locomotion for aquatic animals and snake alike. Their movement is dependent on the lateral and vertical bend of the spine to propel them through the water and along the ground. Limbs for all animals simply act as a pivot point while the spine flexes and bends to maintain balance when the body is in motion (Gracovetsky, 1985).
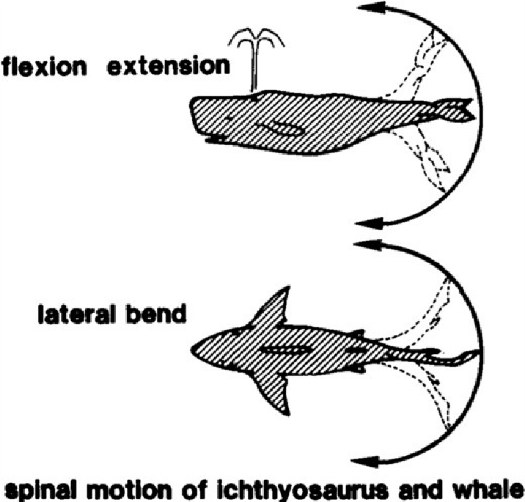
Structural Support
The spine’s main function, from a structural standpoint, is weight distribution. The spinal column forms the central axis of weight-bearing and supports the head as well as transfers the weight of the trunk and abdomen to the legs (DeSai et al., 2020). This allows for the weight of vertebrates to be more evenly distributed, thus meaning that they can move more freely. More differences appear when different mammals are considered. An example would be the difference between how weight is distributed by bipeds and quadrupeds. In bipeds, all the weight is distributed upon two legs, whereas in quadrupeds, the weight can be more evenly distributed across four legs. This has numerous ramifications on the build of each given animal.
A key advantage to having weight distributed across four legs is that more mass can be supported. The heaviest bipeds rarely exceed more than 200 kg, whereas quadrupeds can weigh in excess of 7 000 kg. However, due to their aquatic environment, marine mammals are less affected by gravity in the traditional sense; instead having to deal with water pressure and trying to balance being able to withstand this pressure and buoyancy to ensure that they can dive deep enough to get food, but also be able to swim up to the surface of the water to breathe. Due to their environment, marine mammals can grow to be significantly larger than any land mammals, with blue whales weighing up to 181 500 kg (“Blue whale” | National Geographic, n.d.).
Freedom of Movement
Aside from structural support, another key function of the spine is to facilitate movement. The spine allows for three types of distinct motions, which are extension, rotation, and lateral flexion (Crowell et al., 2020). As such, this allows the body to perform a wide range of motions, such as walking, jumping, bending, and twisting, all distinct motions from one another. Animals with a spine can move around with a lot of freedom of movement. Once again, different constraints and adaptations of different mammals needs to be considered. For example, bipeds, such as apes, have adaptations to better allow for walking with an erect posture, compared to quadrupedal mammals or even marine mammals. One of these differences is the lateral spinal ligaments, which is absent in quadrupedal animals (Jiang et al., 1995). These ligaments help with shortcomings of the bipedal system, such as helping maintain balance, and allowing for easier rotation and flexion of the spine (Jiang et al., 1995). A further distinction is in the type of movements made by different mammals. Quadrupeds, with their four legs, can move a lot faster than bipeds due to their additional legs. While bipeds have many advantages over quadrupeds, speed is not one of them. An additional difference, which must be considered regarding different mammals, is marine mammals. These mammals have different types of movement to those of land mammals due to their aquatic environment. Lands mammals have spines that are optimized for up-and-down movement of running, walking, and, in some cases, jumping movement, whilst allowing for little sideways motion. Comparatively, marine mammals have spines that are more suited for the up-and-down movement of swimming (Jiang et al., 1995).
Shock Attenuation
A consequence of the constant movement and environmental constraints, such as gravity, that the spine is under constant stress. As a result, the spine has developed mechanisms to counteract these stresses. One of the most notable mechanisms is shock attenuation. When moving, each step produces a shock wave that is carried through the body, via the spine, to the head. If these shock waves are not dissipated, it could lead to health problems (Castillo and Lieberman, 2018). The shock waves are dissipated by the lower spine, specifically the lumbar part of the spine (Castillo and Lieberman, 2018). Furthermore, the spine needs to be able to twist and bend; therefore there is no rigid protection around the spine. Aside from the nerves that connect to the spine throughout its entire length, a rigid tube protecting the spinal cord would not be functional. This is because the spine needs to be able to move and flex to withstand any outside forces that it may be subjected to. Overall, the spine has a functionality that lends itself perfectly to the role it must accomplish.
Architecture of the Spine
Vertebrae
Each vertebra of the spine is made up of the vertebral body, the pedicle, the articular processes including the inferior and superior processes, and the spinous process (Jensen et al., 1990; Waxenbaum et al., 2020). The vertebrae are stacked on top of each other with an intervertebral disc separating them. Surrounding this entire structure is a system of ligaments that create more stability and restrict movement to ensure the spine remains within a safe range of motion.

Vertebral Body
This round portion of vertebrae is the most load bearing part of the column (Bogduk, 2005; Jensen et al., 1990; Marras, n.d.) (Fig. 3). The shell of the vertebral body is a layer of very strong cortical bone. Meanwhile, the interior of the vertebral body is made up of cancellous bone otherwise known as trabecular bone (Fig. 4). Trabecular bone is a spongy medium that is more flexible than the exterior shell of the vertebra (Bogduk, 2005). It also creates an interior framework that that helps the bone keep its structure while under stress. The architecture of the trabecular bone can be modeled as a three-dimensional lattice with a unit cell geometry (Jiang et al., 1995). However, the structure is not perfectly columnar and various degrees of disorder. The overall strength of this structure depends on both the architecture and stiffness of the trabecular bone and because the trabecular bone’s spongy texture acts as a series of struts and crossbeams thus enhancing the strength of the body, with most of the stress being put of the trabecular joints (Jiang et al., 1995) (Fig. 5). The shell configuration of the vertebral body allows the vertebra to withstand more dynamic load bearing as opposed to a solid bone (Bogduk, 2005).



Articular Processes
The vertebra also consists of four articular processes attached to the pedicle on the back of the vertebral body. They protrude outside of the bone creating an irregular bone shape (Marras, n.d.). The processes create joints by interlocking with those of the other vertebrae in the column. The inferior articular processes are shaped like hooks that project downward and they attach themselves to the superior articular processes of the vertebra bellow. The joints that join the two processes are known a zygapophysial joint and their interlocking system is a major mechanism that ensures the vertebrae remain in their proper positions as the vertebral body is smooth, has no hook-like features and is only joined by the intervertebral disks. Therefore, the articular processes maintain the alignment of the vertebral column and controls the range in motion with the help of ligaments (Bogduk, 2005). They also help distribute the load when the spine is at certain angles thus making it a weightbearing portion of the vertebra. Finally, the spinous process is a projection and provides a point for the surrounding muscle and ligaments to attach themselves to the vertebra (Bogduk, 2005).
Intervertebral Discs
Between each vertebral body lies an intervertebral disc. The discs are made up of a central nucleus pulposus surrounded by a peripheral anulus fibrosus. The central nucleus is made of mostly water but also contains proteoglycan and collagen (Bogduk, 2005). Meanwhile, the anulus fibrosus is made up of a mix of collagen and elastic fibers and acts as a ligament restricting movement and stabilizing the vertebral column. Therefore, when stress is applied the discs will compress and not break. The disc then is covered by a layer of cartilage on the top and bottom surfaces. The malleable material and structure of the intervertebral discs allow them to be capable of withstanding large weights and transfer the load between each vertebra while being flexible enough to allow the vertebrae to rock without touching one another as seen in Fig. 6 (Bogduk, 2005; Marras, n.d.). The mechanics of the discs allow for the movement required of the vertebral column while maintaining a level of stability which would not be there if the vertebrae were simply stacked on top of each other.

Ligaments
Ligaments run along the posterior and anterior aspects of the vertebral column. They increase the level of stability of the body by resisting excess movement in the vertebra. The anterior longitudinal ligament is made up sets of collagen fibers and they attach to the vertebrae by attaching themselves to the anterior margins of the lumbar vertebral bodies (DeSai et al., 2020). Their purpose is to resist vertical separation of the anterior ends of the vertebral body. Meanwhile, the posterior longitudinal ligaments form a band along the vertebral bodies and expands on the intervertebral discs by attaching its fibers to the annuli fibrosis. The purpose of the posterior longitudinal is to resist the separation of the vertebral bodies since there are not interlinking joints on that side of the vertebral column (Bogduk, 2005).
Mechanics of the Spine
The movement of the spine is considered a system of rigid bodies connected by a series of deformable discs. Each bone in the spine experiences displacement through a series of rotations and translations in the horizontal, sagittal, and frontal planes or more commonly labelled as the X, Y and Z axes (Bogduk, 2005; Marras, n.d.; Panjabi and White III, 1971). definition of these types of movement fall under the fact that translations are movements along the axes while rotations are movements around the axes. There are two main methods to calculate the angle of rotation of the vertebral column (Panjabi and White III, 1971). The first being Euler’s method and the second being the vector method. By Euler’s method using a series of equations the result is three Eulerian angles of rotation of the rigid body (Panjabi and White III, 1971). Meanwhile, when using the vector method, the rotation is seen as a vector therefore is acts as a displacement giving the axis of rotation and the amount of rotation(Panjabi and White III, 1971).

The vertebral column undergoes stress and strain under the guise of tension and compression. These forces are put on the spine due to weight and general movement. The structure of the vertebral column is an interesting feat of engineering as it is not a straight line. Instead, it has three curves that create an S-shape. The curvatures occur at the cervical, thoracic, and lumbar sections of the spine as shown in Fig. 8. This curvature increases the spine’s ability to withstand a greater amount of stress by distributing the weight (Betts et al., 2013). By doing so it improves the overall strength, flexibility, and ability to absorb shock of the spine.

Presentation of the Brittle Star
Brittle stars are a marvel of biological advancement. The brittle star is an astounding creature as it has managed to survive and thrive without brain or a spine. This animal is one member of an entire family of anatomically similar creatures. More specifically it is one of thousands of animals known as echinoderms (Fig. 9). The latter are defined most distinctly by their anatomical symmetry . The phylum Echinodermata is made up of approximately 7 000 species making it a large and varied phylum (“Echinoderms” | Marine Education Society of Australasia, 2015). Echinoderms can be found at any shapes, any sizes and any region or depth of the ocean. Furthermore, echinoderms are a spin on the classification Echinodermata rooting from the Greek words akenos which translates to Hedgehog and derma, the Greek word for skin (“Echinoderms” | Marine Education Society of Australasia, 2015).

Looking at a brittle star (Fig. 10), it is evident to see why the phylum was named the way it is. Like many other echinoderms, brittle stars are host to bumpy, rigid flesh that gives them a rugged appearance (“Marine Invertebrates: Brittle Stars (Ophiuroidea)” | Canada’s Artic, n.d.). However, beyond their spiny looks, there is a more integrate feature to the classification of the echinoderms that makes them what they are and that can be rooted back to the basis of their physiological makeup. In biology, bilateral symmetry is the notion that an organism’s body can be divided into halves, present in more than 99 % of all animals, including humans. Nevertheless, the specificity of echinoderms is that they do not present bilateral symmetry as they have five sides to their symmetrical anatomy called Pender radial five-sided symmetry (“Marine Invertebrates: Brittle Stars (Ophiuroidea)” | Canada’s Artic, n.d.).

Just like many echinoderms, the brittle star generally has five long articulated arms (that can reach up to 60 cm in length for the larger specimen) which allow them to crawl across the sea floor. These arms are flexible, completely independent of the body and do not touch at their base, which distinguishes them from Asterias (starfish) (“Marine Invertebrates: Brittle Stars (Ophiuroidea)” | Canada’s Artic, n.d.).
Design Commonalities between the Brittle Star and the Mammalian Spine
The arms of brittle stars are joined at a central disk (as can be seen on Fig. 10) that contains their vital internal organs. Furthermore, brittle stars can detach one of their arms if they are attacked by a predator. Their body is supported by an internal endoskeleton of calcium carbonate plates that covers all the disk and the five arms (“Echinoderms” | Marine Education Society of Australasia, 2015).
Looking at the anatomy and the skeleton (Fig. 11.), it can be seen that each segment contains calcareous skeletal elements which includes the central vertebral ossicle associated with four peripheral arm shields: a dorsal, a ventral and two lateral ones (surrounded by spines) (Zueva et al., 2018). As previously discussed, instead of having a brain, echinoderms possess a central nervous system (called a nerve ring) that acts to coordinate the movements of its arms. The nerve ring is connected to the radial nerve cord (present in each arm) and protected by the oral skeletal shield and oral ligament (Zueva et al., 2018).

Normally, the arm spine joint of O.nigra is a mobile diarthrosis (i.e., an articulation that permits free movement) and act as a flexible joint in which the articular surfaces of the spine base and lateral plate tubercle (which is a small, rounded projection on bone) are separated. In some cases, the erect spines may become completely immobile. If this occurs, the joint will function as a rigid synarthrosis (it means that the joint allows no movement) (Wilkie, 2016).
The movements of the spine (and therefore of the brittle star) are facilitated by the presence of circumferential rides in the epidermis which surrounds the joint but also by the interarticular connective tissue which makes it possible to reduce frictional forces between the articular surfaces. The inclination of the spine comes down lonely to the action of forces external to the joint and the re-erection of the inclined spines is achieved partly by the elastic recoil of the ligament of the spine but also by the contraction of the muscle of the spine (Wilkie, 2016).
The role of juxtaligamental nodes (Fig. 12.) in the functioning of the joints is to allow the direct modulation of the tensile properties of the ligament of the spine by their neurosecretory cells (which are cells that have the capacity to secrete neurohormones, whose function is to translate neural signals into chemical stimuli). In addition, the ultrastructural features (which are the biological structure of a cell that is not visible through an ordinary microscope) of the nodes strongly suggest that they have an integrative role and that they can coordinate the spinal joint effector system (Wilkie, 2016).


To conclude, the experiments used may help demonstrate the affirmations above on the operation of the arms of a brittle star and the connections established with the spine of a vertebrate were made on the brittle star O.nigra. However, it is reasonable to assume that everything above can be largely applied to all the arm spine joints of all ophiuroids that present the same spine base and tubercle morphology as that of O.nigra which is the case for most species (Zueva et al., 2018).
First, it is obvious that these two organs still have some differences. The most important one is the location as well as the shape it takes since the vertebrates have a single vertebral column while the echinoderms have five arms. In vertebrates, the spine contains the spinal cord directly connected to the brain while, in echinoderms, the cord nerves (could be assimilated to the spinal cord) are not connected to the brain but to a ring nerve (“Brittle star” | New World Encyclopedia, n.d.). Moreover, there are even more observable differences.
For instance, given enough time, sea stars can grow back one of their arms if it has been damaged, harmed or removed. Moreover, for some species, the severed arm can grow back into a complete sea star. However, for most sea stars, a severed limb dies. It is not the case in vertebrates, since the spine is included in the body; a severed spine will not grow back and could cause several damages to the body, especially if the spinal cord is affected. Moreover, it has be observed that the echinoderm can reject one of its arms to defend itself from predators (Wilkie, 2016).
Application of the Spine in Engineering
The spine has inspired many different engineered structures throughout the world, but the most notable one is bridges. Bridges, like the spine, are designed to bear an incredible amount of weight, and they do this by distributing across the center outwards. Overall, the spine can be used to improve many engineering structures and it needs to be studied more to help improve the modern world.
Conclusion
The spine is one of the most essential organs in vertebrates. It is made up of a sequence of vertebrae each separated by intervertebral discs. These two make up the vertebral column that supports the entire body of vertebrates and allows for movement (Betts et al., 2013). The spine allows balanced weight distribution and facilitates body movements. To counteract the stresses faced, it performs shock attenuation. In addition, it protects the spinal cord, an essential cable of nerves that carry messages from the brain to the rest of the body.
Each vertebra is made up of the vertebral body, the pedicle, the articular processes including the inferior and superior processes, and the spinous process (Bogduk, 2005; Waxenbaum et al., 2017). The vertebrae are stacked on top of each other with an intervertebral disc separating them. Surrounding this entire structure is a system of ligaments that create more stability and restrict movement to ensure the spine remains within a safe range of motion. The structure of the vertebral column is a marvelous feat of engineering. It is not a straight line; instead, it has three curves that create an S-shape. This curvature increases the spine’s ability to withstand a great amount of stress by distributing the weight (Betts et al., 2013). Consequently, it improves the overall strength, flexibility, and ability to absorb the shock of the spine.
In evolutionary biology, convergent evolution is the process whereby organisms not closely related (i.e., not monophyletic) independently evolve similar traits as a result of having to adapt to similar environments or ecological niches. On a molecular level, this can happen due to random mutation unrelated to adaptive changes (Waxenbaum et al., 2017).
The brittle star is an echinoderm that is an invertebrate. Though it does not have a spine, its mechanisms closely relate to the function of one. By looking at these similarities, insight can be gained into the mechanisms of this key body part, which can then be applied to technology in the real world.
Echinoderms possess a nerve ring that acts to coordinate the movements of their arms. The nerve ring is connected to the radial nerve cord (present in each arm) and protected by the oral skeletal shield and oral ligament. Thus, it can be seen that it is one of the characteristics, which is also present in vertebrates: the main purpose of the spine’s design is to protect the nervous system.
The movements of the spine (and the brittle star) are facilitated by the presence of circumferential rides in the epidermis, surrounding the joint. They are also facilitated by the interarticular connective tissue, which makes it possible to reduce frictional forces between the articular surfaces. The inclination of the spine comes down lonely to the action of forces external to the joint and the re-erection of the inclined spines is achieved partly by the elastic recoil of the ligament of the spine but also by the contraction of the muscle of the spine.
There are many differences as well. The most important one is the location as well as the shape it takes. In vertebrates, the spine contains the spinal cord directly connected to the brain whereas, in echinoderms, what could be assimilated to the spinal cord (i.e., the cord nerves) is not connected to the brain but a ring nerve.
References
Artic, C. s. (n.d.). Marine Invertebrates: Brittle Stars (Ophiuroidea). Retrieved from http://www.arctic.uoguelph.ca/cpl/organisms/inverts/marine_inverts/ophiuroids.htm
Australasia, M. E. S. o. (2015). Echinoderms. Retrieved from http://www.mesa.edu.au/echinoderms/
Betts, J. G., Young, K. A., Wise, J. A., Johnson, E., Poe, B., Kruse, D. H., . . . DeSaix, P. (2013). The Vertebral Column. In Anatomy and Physiology. Retrieved from https://openstax.org/books/anatomy-and-physiology/pages/7-3-the-vertebral-column
Bogduk, N. (2005). Clinical Anatomy of the Lumbar Spine and Sacrum: Elsevier/Churchill Livingstone. Retrieved from https://books.google.ca/books?id=UYC_NpoFfAsC
Castillo, E. R., & Lieberman, D. E. (2018). Shock attenuation in the human lumbar spine during walking and running. Journal of Experimental Biology, 221(Pt 9). doi:10.1242/jeb.177949
Crowell, M. G., Rahmat, S., & Koretsky, I. (2020). Correlation of Bone Density in Semi-Aquatic and Aquatic Animals with Ecological and Dietary Specializations. The FASEB Journal, 34(S1), 1-1. doi:https://doi.org/10.1096/fasebj.2020.34.s1.01860
DeSai, C., Reddy, V., & Agarwal, A. (2021). Anatomy, Back, Vertebral Column. In StatPearls. Treasure Island (FL): StatPearls Publishing
Encyclopedia, N. W. (n.d.). Brittle star. In New World. newworldencyclopedia.org. Retrieved from https://www.newworldencyclopedia.org/entry/Brittle_star
Geographic, N. (n.d.). Blue whale. Retrieved from https://www.nationalgeographic.com/animals/mammals/facts/blue-whale
Gracovetsky, S. (1985). An hypothesis for the role of the spine in human locomotion: a challenge to current thinking. Journal of Biomedical Engineering, 7(3), 205-216. doi:10.1016/0141-5425(85)90021-4
Jensen, K. S., Mosekilde, L., & Mosekilde, L. (1990). A model of vertebral trabecular bone architecture and its mechanical properties. Bone, 11(6), 417-423. doi:10.1016/8756-3282(90)90137-n
Jiang, H., Moreau, M., Raso, V. J., Russell, G., & Bagnall, K. (1995). A comparison of spinal ligaments–differences between bipeds and quadrupeds. Journal of Anatomy, 187 ( Pt 1)(Pt 1), 85-91. Retrieved from https://www.ncbi.nlm.nih.gov/pmc/articles/PMC1167351/
Marras, W. S. (n.d.). Biomechanics of the Spinal Motion Segment. In. musculoskeletalkey.com. Retrieved from https://musculoskeletalkey.com/biomechanics-of-the-spinal-motion-segment/
OrthoInfo. (n.d.). Spine Basics. Retrieved from https://orthoinfo.aaos.org/en/diseases–conditions/spine-basics/
Panjabi, M., & White, A. A. (1971). A mathematical approach for three-dimensional analysis of the mechanics of the spine. Journal of Biomechanics, 4(3), 203-211. doi:10.1016/0021-9290(71)90005-4
Waxenbaum, J. A., Reddy, V., Williams, C., & Futterman, B. (2020). Anatomy, Back, Lumbar Vertebrae: StatPearls Publishing, Treasure Island (FL). Retrieved from http://europepmc.org/abstract/MED/29083618
Wilkie, I. C. (2016). Functional Morphology of the Arm Spine Joint and Adjacent Structures of the Brittlestar Ophiocomina nigra (Echinodermata: Ophiuroidea). PloS One, 11(12), e0167533-e0167533. doi:10.1371/journal.pone.0167533
Zueva, O., Khoury, M., Heinzeller, T., Mashanova, D., & Mashanov, V. (2018). The complex simplicity of the brittle star nervous system. Frontiers in Zoology, 15(1), 1. doi:10.1186/s12983-017-0247-4