A Biochemical Analysis of Mammalian Milk: Diversity Amongst Species and Biomedical Applications
Zachary Gurberg, Olivia Clague, Ayoub Rabhi, Di Ah Lim
Abstract
Lactation is a natural phenomenon that unifies the mammalian class and serves the fundamental purpose of supplying offspring with the essential nutrition and immune protection for survival in the early stages of life. Despite the existence of physiological diversity across lactating species, the general biochemistry of mammalian milk is a common factor of all lactating species. The biochemistry of the various components of mammalian milk will be investigated further with reference to the synthesis of vital nutrients as well as the chemical composition of the various fractions of milk. Furthermore, the stages of lactation will be discussed to highlight the fluctuations of the concentrations of the components of mammalian milk throughout the lactation process. Additionally, an exploration of the primary constituents of milk will be detailed in order to examine the fundamental purpose of these components for offspring development. Following the investigation of the consolidating biochemical processes and composition of mammalian milk, diversity present amongst various species will be emphasized and explained in reference to differing selective pressures and offspring nutritional requirements.
Introduction
The process of lactation is a defining trait for mammalian species as it lays the foundation for neonate survival due to fundamental nutrients and components supplied by the milk. The importance of mammalian milk is apparent as milk provides offspring with essential nutrients such as lipids, proteins and carbohydrates that facilitate their development and immunity. In addition, the examination of the biochemical synthesis and composition of mammalian milk is vital to the analysis of unity and diversity observed amongst mammalian species. The exploration of the general components of mammalian milk illustrates the nutritional and immunity requirements shared amongst mammalian offspring in order to survive and develop in the early stages of life. Furthermore, the investigation of the differences in milk composition amongst species highlights the impact of differing selective pressures species face as well as the specific nutritional and immune demands of various mammalian offspring. Moreover, the exploration of the biochemical similarities and differences of milk composition amongst the mammalian class provides further insight into the complex evolutionary pathways that have resulted in the mammalian species that are present today. Finally, as bioengineers, we can seek to harness the unique traits of mammalian milk for various applications in the medical world.
General Biochemistry of Milk in Mammals
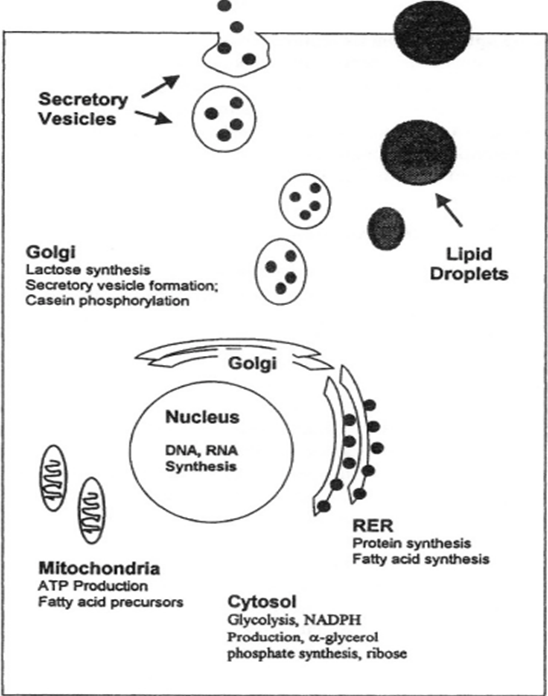
In order to properly understand the interesting biochemical differences in the composition of mammalian milk, it is important to first recognize the commonalities of milk in all species. In all mammals, milk is synthesized in the mammary glands from the building blocks available from circulating blood [1]. This is done by means of transport vesicles that adhere to more general hormonal regulation taking place before/after and during pregnancy. The main components extracted from the blood for milk biosynthesis are amino acids, glucose, fatty acids, and minerals [2]. This extraction step is crucial because these components are critical precursors to the nutrients that will be provided to the young offspring, and some of these nutrients extracted from the blood will pass directly to the newly synthesized milk without further modification by the mammary epithelial cells [2].
Mature milk is composed of three general fractions: water, fatty fractions, and non-fatty fractions [1]. The water fraction is composed of the general milk serum proteins such as casein and albumin. These proteins are generally synthesized de novo in the mammary epithelial cells by the rough endoplasmic reticulum and the Golgi apparatus. The fatty fractions contain the major fatty molecules, mostly triglycerides and fatty acids. These fatty acids are generally synthesized in the RER and the mitochondria [2], though the specific fatty acids synthesized are highly variable species-to-species. Finally, the non-fatty fractions consist of many different components such as nitrogenous substances, carbohydrates, and water-soluble vitamins and minerals. Some of these components are synthesized de novo, whereas others pass directly from the blood to the milk through the mammary epithelial cells [2]. Although all mammals' milk will contain these key elements, the specific proportions of each component are highly variable depending on the species, as the composition is a direct response to the environment in which the species lives. Furthermore, the proportions can change from individual to individual depending on genetics, age, physique, frequency of lactation, and most importantly, stage of lactation [1].
The Stages of Lactation
The biosynthesis of milk, just as the growth of mammary tissue, is a response to hormonal changes that happen inside the body. Mammary tissue is developed far before gestation, but the tissue remains in a dormant state, meaning that the feeding parent does not actively produce milk if there is no need to. However, if conception occurs, mammary tissue continues to develop through pregnancy with accelerated rapid tissue growth [3]. This is regulated by basic endocrine hormones such as oxytocin, progesterone, and estrogen among many others [3].
While the circulatory levels of these hormones remain high during pregnancy and up to one week following parturition, the feeding parent produces colostrum; a very different feed for the infant than mature milk. Colostrum has a far higher sugar and immunoglobulin content than mature milk, and very low amounts of fat. This is because the intestinal tract of the newborn has not yet been colonized by bacteria [3]. Thus, the colostrum provides the intestinal tract with the proper environment for the colonization of bacteria while inhibiting the reproduction of pathogens. Furthermore, the colostrum provides the infant with its first source of antibodies, effectively priming the digestive tract for mature milk, which is a lot more complex in composition [3].
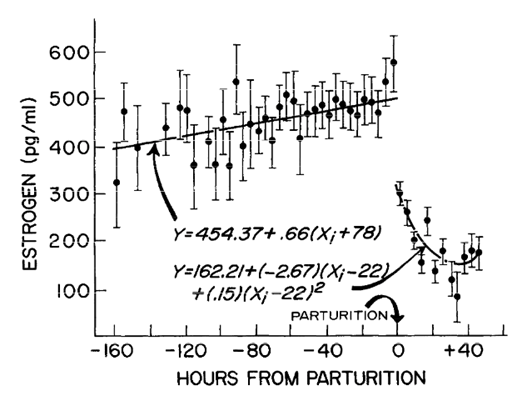
Following the expulsion of the fetus, the levels of progesterone and estrogen in the body drop significantly [5]. This causes the mammary glands to transition from the production of colostrum to the production of mature milk, which will now be regulated by the frequency of lactation [3]. In the early stages of infancy, lactation occurs frequently, thus demanding that the mammary glands produce a significant amount of milk. However, once the infant matures and relies more on solid food, the lactation occurs less frequently and thus the milk production slows. This causes the later mature milk to have less water content and thus have a higher concentration of other compounds [3].
It is thus clear to see that the concentrations of the various components in milk change depending on the needs of the feeding infant. This makes mammalian milk a very versatile and interesting “superfood”, where all the components play a distinct role, ripe for analysis.
“Diving” into the Components of Mammalian Milk
Lipids
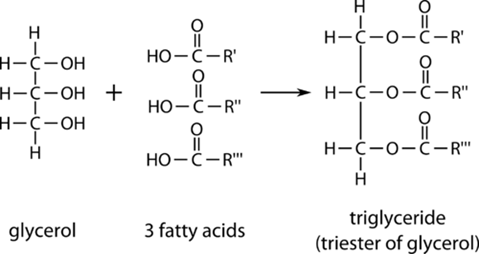
Lipids are the most variable out of any of the major constituents of mammary milk [2]. They are composed almost entirely of triglycerides (98%) and fatty acids, but there is a high degree of variation in terms of the specific fatty acids and respective concentrations from species to species as well as individual to individual [2].
On an individual basis, the concentration of lipids can depend highly on the stage of lactation, as shown by the low levels of fat in colostrum, but it can also depend highly on the periodicity of feeding. Fat is the most energy-dense compound of milk, so as feeding becomes less frequent, the fat content subsequently increases to meet the daily energetic demands of the young. The fatty acids present in mammalian milk originate in three distinct ways [2]:
- From glucose conversion in the cytoplasm of mammary cells
- From the diet through the hydrolysis of chylomicron, a lipoprotein rich in triglycerides [6]
- From de novo synthesis in the mammary cells from other more rudimentary components.
The concentrations of various fatty acids differ in each species, and each fatty acid will originate in one of these three ways. Typically, shorter fatty acids are more likely to come from de novo synthesis, whereas longer ones typically come from the diet [2].
Proteins
Proteins are an integral component of mammal milk as they provide crucial amino acids that promote both the growth and development of offspring in the early stages of life [8]. Milk proteins are synthesized from amino acids that originated from either the bloodstream or from amino acids synthesized by the secretory cells of the mammary gland [2]. The primary proteins found in mammalian milk are caseins and whey proteins as well as immunoglobulins, enzymes and lactoferrin [10]. Caseins constitute 80% of milk proteins and not only supply essential amino acids to neonates but also play a pivotal role in the milk digestion process [10,2]. Caseins are responsible for the gradual delivery of milk nutrients to the intestine which is vital for species that feed less frequently, such as rabbits, as nutrients are slowly absorbed in between feedings to maintain cellular and bodily processes [10]. The casein protein component of milk is made of various derivative of casein including aS1-casein, aS2-casein, B-casein and k-casein. The functional properties differ for each casein component since they have a primary amino acid sequence and therefore provide offspring with various essential amino acids [8]. Furthermore, the casein proteins and calcium phosphate form structures called micelles which are large colloidal particles that contribute “fluidity to casein molecules” in addition to dissolving calcium and phosphate (Figure 5)[8]. In addition, whey proteins are an important component of mammalian milk as they play a role in lactose synthesis, passive immunity, vitamin A transfer and fatty acid transfer [9]. Furthermore, immunoglobulins play an essential role in providing passive immunity to offspring which is especially important for ruminants as there is no immunity obtained by the fetus in utero [10].

The total protein concentration in mammalian milk as well as the amount of the various milk proteins varies between species due to several factors. The “law of lactation” predicts the growth rate of a specific mammal's offspring in the early stages of postnatal life by examining the time required for the offspring to double its body weight [7,1]. The “law of lactation” is directly related to the protein content of the specific species of milk and ultimately suggests that lower the protein concentration in milk, the more time it takes for the offspring to double its body weight. There are some exceptions however, as milk of low protein concentration is typically observed in species that feed their young frequently [1]. An example of this is mice as they feed their offspring many times per day and therefore have lower protein content in their milk [1].
Carbohydrates
Lactose is generally the primary carbohydrate present in mammalian milk and functions to supply energy to the offspring as well as promote the absorption of calcium in the gut [9]. Lactose is a primary energy source for most mammalian young since it's a disaccharide that is composed of the monosaccharide's galactose and glucose (Figure 6) and therefore produces twice as much energy compared to that of a single monosaccharide [10]. Furthermore, lactose is an interesting component as it is synthesized exclusively in the mammary gland via the enzyme lactose synthetase. Lactose also has an influence on milk secretion as high concentrations of lactose in the secretory vesicles is responsible for osmotic swelling in the vesicles, ultimately dictating the volume of milk secreted from the mammary gland [10,12].
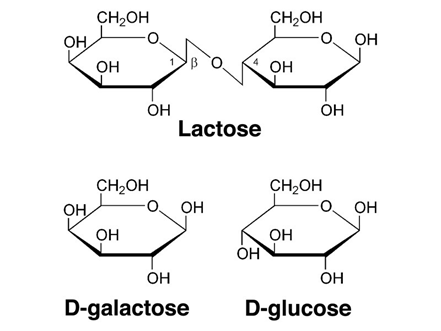
Supplementary to the primary carbohydrate lactose, small quantities of other carbohydrates such as glucose, galactose and a few nitrogen-containing carbohydrates are present in mammalian milk [1]. The disaccharide galactose plays a significant role in the biosynthesis of complex carbohydrates, glycoproteins, and glycolipids. That's why the disaccharide galactose is a very important component of mammal milk [12].
The lactose concentration in mammalian milk varies across species with primates having around 7% lactose concentration compared to marine mammals that have only trace amounts of lactose or none at all [1,2]. Some species, such as marsupials, produce milk that contains large oligosaccharides that increases the carbohydrate of the milk and also enables the milk to have a higher concentration of other active constituents such as electrolytes [11]. The significance of this diverse marsupial milk composition is from a nutritional standpoint as marsupial offspring require a higher concentration of carbohydrates and electrolytes for greater energy and nutritional support in the early stages of life [11].
For mammals that have lactose present in their milk, unlike the protein and lipids components of milk, the lactose content is not influenced by diet and stays relatively stable throughout the lactation process [1].
Diversity of Mammalian Milk Amongst Various Species
Although all mammalian milk is composed of the same basic nutrients, the precise constituents and concentrations of these various nutrients depends on the species in question. The milk of certain mammals has evolved in spectacular ways due to diverse selective pressures, giving the young the best chance at survival in their distinct environment. Additionally, as bioengineers we can draw inspiration from these unique adaptations in terms of biomedical applications.
Monotreme
The monotremes are a subclass of mammals that have a unique composition of milk compared to that of other mammalian species. The milk of monotremes is primarily composed of a protein called monotreme lactation protein (MLP), which is especially expressed in the milk cells. The monotreme lactation protein is an alpha-helical protein, which has the unique characteristic of possessing antimicrobial properties. This protein impales bacteria through the utilization of its unique shape in the form of ringlets, effectively killing them. This protein likely evolved centuries ago in order to protect hatchlings from dangerous bacteria ingested while suckling milk at the surface of the nursing monotreme's skin. This protein is necessary for monotremes because they have no nipples and thus the young drink the milk from the skin surface where many bacterias reside.[13]
Echidna

One such example of a monotreme is the echidna, also known as the anteater (Figure 7). Echidna's milk contains a high concentration of lipids which are 95% triglycerides. This makes their milk exceptionally thick. Furthermore, the echidna's milk contains a characteristically high concentration of proteins, carbohydrate components and some minerals. This milk is very rich and is a good source of nutrients, which can be explained by the size of the young. The young are born underdeveloped and need a very high concentration of nutrients to grow, due to their distinctly high rate of growth [15].

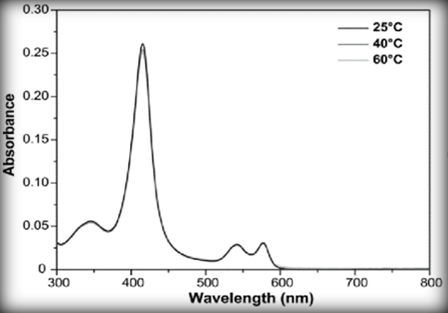
What is most distinct about the milk of this monotreme is that it has a very high concentration of iron. This iron comes from the blood of the female echidna in the form of hemoglobin. In fact, there is so much iron in the milk that the colour of the milk is pink, distinguishing it from other mammalian milk that is white/cream in colour. Each hemoglobin has four nitrogens in the center of the molecule (Figure 8), which forms a bond with the iron (Fe). The role of this protein is to interact and transport oxygen everywhere in the body of the animal. [16]
The bond between oxygen and the iron can reflect light. If we analyze the UVs spectroscopy of the hemoglobin (Figure 9) that has interacted with oxygen, we can see that the molecule absorbs most blue-green light. It means that the molecule will reflect a red light. So, these iron binding proteins are red, and if mixed up with a white milk, it will result in a pink milk.[17]
It is important for the female echidna to give hemoglobin to the young because the young have a low blood concentration of hemoglobin at birth. This can be explained by the fact that the young are too underdeveloped to produce hemoglobin. So, to fix this problem, the echidna opted for a strategy where the female gives hemoglobin to the young via lactation. The concentration of iron in the milk will decrease as the young grows and as they are able to produce hemoglobin. [15]
Marsupials
The Tasmanian devil
Another predominantly studied group of mammals are the marsupials. Generally, the young of marsupials are born underdeveloped and don't have a good innate immune system. To remedy that, the mother produces a milk that is rich in proteins and antibodies to help with the growth and the immune system of the young.[18]
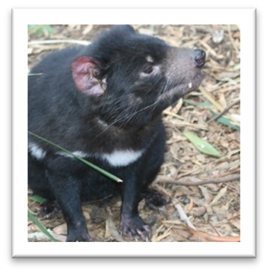
The Tasmanian devil is an example of a marsupial (Figure 10). Their milk also plays an important role in helping their young combat bacteria and infections in early development, much as with the monotremes. This is done through a high concentration of macrophages, lymphocytes, and neutrophils in their milk, but in particular, it is the protein content of their milk that holds the strongest antimicrobial properties [20]
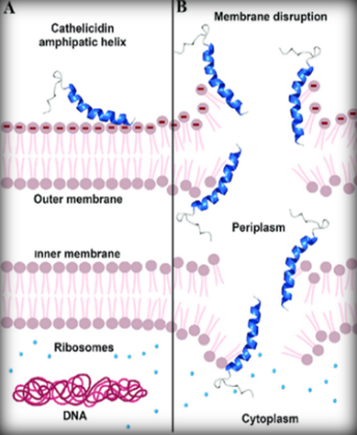
The milk of the Tasmanian devil is composed of proteins that help with the growth of the young, but it also contains a certain protein called the early lactation protein (ELP). This protein plays an important immune role. The proteins in the milk of the Tasmanian Devil are made up of six different peptides, each composed of 120-171 amino acids that have an amino group and a carboxyl group.[18] Out of these six peptides, three seem to have antimicrobial properties. These antimicrobial peptides are called cathelicidins. The peptide is a molecule charged positively (amino group) and attacks the membrane cell of the bacteria that is charged negatively (Figure 11). This will form a hole in the membrane of the bacteria and all its cytoplasm will be drawn out. This will automatically result in death for the bacteria.[22]
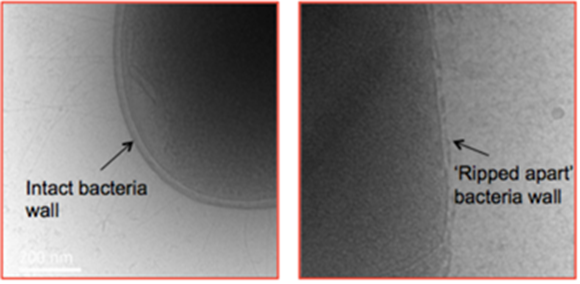
Out of the three antimicrobial peptides, two (Saha-CATH5 & 6) are capable of killing bacteria that are known to be harmful to humans. Studies have shown that these peptides can even kill deadly superbugs, a sort of bacteria that is immune to antibiotics. The third type of peptide, Saha-CATH3, is effective against certain types of fungi that infect the human lungs and the central nervous system.[22] These fungi and superbugs could potentially kill millions of humans and utilizing these peptides could be an important tool in combating these deadly infections. However, these animals are going extinct, and so milking the Tasmanian devil just to have these antimicrobial peptides could be deemed unethical. Thus, bioengineers and biomolecular engineers have been trying to create artificial peptides that will mimic the properties of the peptides of this marsupial by doing all sorts of synthesis. For now, they found a way to create a star shaped peptide that can rip the membrane of certain bacteria (figure 12), but further research is being done to establish whether or not this could be useful for medical applications [23].
Tammar Wallaby

The tammar wallaby is a marsupial that can be found in low bushes, coastal areas, and sclerophyll forests of Australia. In addition, their diet primarily consists of bark and plants found in grassland regions [24] (Figure 13). Their milk is distinct compared to other mammalian milk as it has the richest sugar content among mammals with sugar constituting 14% of the total nutrients present in the milk itself. Another distinct characteristic of the milk produced by tammar wallaby is that oligosaccharides constitute most of the carbohydrate component of their milk, while lactose is the predominant source of sugar in eutherian milk [25,26]. Oligosaccharides are rich in the milk of the tammar wallaby as they function to kill microorganisms such as bacteria in the mammary epithelial tissue. Also, oligosaccharides serve a function of maintaining the mammary gland during a long lactation period [27,28]. While lactose is a disaccharide composed of two monosaccharides (galactose and glucose), oligosaccharides are structurally diverse as they are composed of only a few monosaccharide units [29,30] (Figure 14 and 15). In addition, the importance of oligosaccharides in the milk produced by the tammar wallaby is illustrated by the reliance of offspring to obtain higher concentrations of sugar and energy in the early stages of life. This increased sugar content is especially vital for the proper development of the immature organs of neonates, particularly the forestomach [27].
The forestomach is a unique organ where microbes are cultured that function to aid in the efficient digestion of complex sugars and fibres obtained from their diet and plays a role in altering the concentrations of each component in the milk [31]. As a result of the increased sugar component of their milk, the milk itself is relatively easy to digest as sugars are easier to digest than fat [32]. It is particularly necessary for premature joeys to obtain enough nutrients from the milk to not only ensure their survival but accelerate the joey's physical growth rate [33,34].
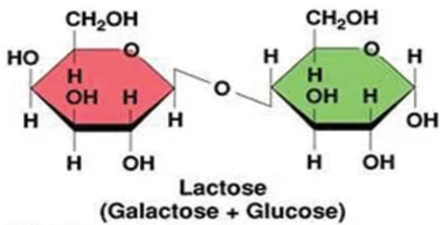

Eutherians
Whales
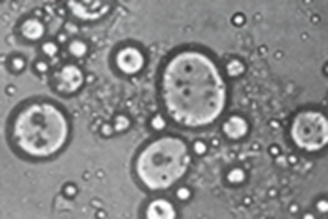
Whales belong to a subclass of marine mammals called Cetaceans and inhabit all the major oceans. Whales' milk is unique due to the fact that it is composed of 35- 50% of lipids components. This diverse composition of the lipids contained in the milk consequently makes whales' milk the most highly concentrated mammalian milk in terms of lipids. In addition, their milk contains high levels of protein and low levels of lactose which is illustrated in the figure 16 and 17 [35]. As seen in figure 16, the milk cells are large, resembling the size of fat globules which suggests a higher fat content than typical mammalian milk [36].

The reason why whales' milk has the highest fat concentration compared to other mammals can be explained by the reliance of whales on lipids for survival. The energy provided by the lipid content of their milk is essential as whales inhabit cold water in addition to swimming long distances at high speeds regularly. Lipids provide high amounts of energy and heat which helps combat the cold conditions in a way that other components such as carbohydrates and proteins cannot [35]. In addition, the high-fat content results in an increase in the surface tension and thickness of their milk, resulting in a consistency similar to that of ice cream. The greater surface tension of whales' milk can be explained by the interactions between the lipid components and the water dispersed in the milk itself. The lipids found in mammalian milk are composed of fatty acids that have a long non-polar hydrocarbon chain and a polar carboxyl group [37,38] (Figure 18 and 19). In mammalian milk, the polar heads of fatty acids attract both polar water molecules and other polar heads of fatty acid molecules. Consequently, the high fat concentration of whales' milk results in stronger intermolecular forces within the milk, thus resulting in a higher surface tension as the greater intermolecular forces, the greater the surface tension [39]. The surface tension of whales' milk gives rise to the lactation mechanisms of whales as the milk is able to pass through the water without dissolving, enabling the calf to obtain the necessary nutrients from the milk [40]. If the fat content of their milk was low, the milk would have greater fluidity, thus it would be diluted into water, preventing the calf from directly obtaining the nutrients from its mother. Moreover, the weight and body size of whales are the biggest among mammals, providing insight into the importance of the lipid content in the milk that calves require as enough nutrients to grow and gain weight faster than other mammals [41].

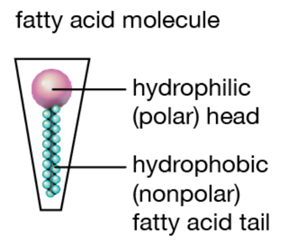
Eastern Cottontail rabbit
The eastern cottontail rabbit is an herbivore and therefore obtains essential nutrients from plants and vegetables. It inhabits various environments like grasslands, forests and mountainous areas. The most distinct feature of its milk is that its protein component has the highest concentration amongst all mammalian milk, with protein constituting roughly 15% of the milk constituents [42] (Figure 20). In comparison to whales, the composition of rabbits' milk is very similar as it has a high lipid concentration in addition to low levels of lactose [43].

A few factors can provide explanations as to why rabbit's milk is the richest mammalian milk in terms of protein content. Firstly, the nursing behavior of rabbits is infrequent compared to that of other mammals as the young are separated from their mothers for longer periods of time. Therefore, it is essential for rabbit's milk to be quite concentrated in terms of nutrients to aid the rapid growth and development of offspring [33]. Increasing the protein content during lactation provides several biological advantages to the young rabbits to ensure their survival as vital nutrients are obtained despite infrequent feedings. Among all protein components in the milk, arginine is the predominant amino acid present in rabbit's milk. Arginine is a positively charged molecule that can bind to phosphate, sulphate, and carboxylate groups to balance the overall charge of the protein. Positively charged carbon cations in the guanidino head group can connect to negatively charged phosphorus, sulfur, and carbon anion via hydrogen bonds [44] (Figure 21). Arginine has a significant effect on body weight gain and physical growth in short periods of time, making this an essential component of rabbit's milk. In addition, arginine plays a central role in the maintenance of the immune system as well as mammary tissues [43,45]. Furthermore, rabbits have a high metabolic rate and have evolved a better digestive system compared to other herbivores which permits young rabbits to digest milk with high energy and protein content, which in turn facilitates the growth and survival of young rabbits [46].
Conclusion
Lactation is one of the unique and defining traits of the mammalian class, serving a plethora of functions that help mammalian young survive early development. All mammal milk consists of the same basic components, synthesized in or passing through the mammary epithelial cells, that provide essential nutrition to the young. Lipids are the main energy provider of milk, being the most energetically dense nutrient. These lipids are mostly in the form of triglycerides, but several fatty acids are present in the milk as well. Lactose, synthesized exclusively in the mammary cells, is the main carbohydrate present in milk. It serves the function of promoting calcium absorption in the gut as well as providing simple energy. Proteins, responsible for the growth and development of the young, provide essential amino acids that act as the building blocks for new biostructures, adhering to the “law of lactation”. Proteins also serve an important function in terms of providing immunity to the newborn, as mammalian newborns are born without antibodies.
The specific concentrations of these components vary generally by the stages of lactation, which are directly related to hormonal concentrations in the blood of the nursing parent. In the early stages of lactation, the nursing parent produces colostrum, a sort of immature milk, as a response to high concentrations of estrogen and progesterone in the blood. These hormonal concentrations drop significantly in the days following parturition, and the nursing parent begins producing mature milk, that is defined differently in each species. Using the same basic components, various species adapt their milk in spectacular ways in order to beat natural selection and survive in their respective environments.
The Echidna's milk contains important proteins such as the monotreme lactation protein which possesses unique antimicrobial properties. Furthermore, the echidna's milk has a distinct pink colour, due to the high concentrations of hemoglobin present as a response to the young's innate inability to produce this important protein. The Tasmanian devil is another example of a monotreme with unique milk properties. An endangered species, the Tasmanian Devil produces milk containing three incredibly powerful antimicrobial peptides. These peptides have the capability of eliminating highly dangerous bacteria and fungi and are being studied as a potential weapon against the inevitable apparition of a superbug.
Furthermore, the Tammar Wallaby milk has the highest concentration of sugars, due to the young's need to develop a unique organ known as the forestomach. This is in striking contrast with the Whale, whose milk has the highest known concentration of fat. This high concentration of fat provides the infant whale with the energy needed to swim long distances, but it also allows the milk to have enough surface tension to pass through water without dissolving. Finally, the Eastern Cottontail Rabbit's milk has the highest concentration of proteins, as explained by infrequent feedings contrasting with a distinctly high rate of growth for the young.
Thus, mammalian milk is not only a characteristic trait defining the mammalian class, but it also provides a unique outlook for potential research. As bioengineers, we can seek to replicate, modify, or reproduce the various unique milks of the animal kingdom in order to create superfoods, healing agents or even antibiotics.
References
1. International Atomic Energy Agency, World Health Organization, & Iyengar, G. V. (1982). Elemental composition of human and animal milk (IAEA-TECDOC–269). International Atomic Energy Agency.
2. Akers, M. R. (2002). Lactation and the Mammary Gland (1st ed.). Wiley-Blackwell. https://doi.org/10.1002/9781119264880
3. Hinde, K., & Milligan, L. A. (2011). Primate milk: Proximate mechanisms and ultimate perspectives. Evolutionary Anthropology, 20(1), 9–23. https://doi.org/10.1002/evan.20289
4. Rouse, B. T., & Ingram, D. G. (1970). The total protein and immunoglobulin profile of equine colostrum and milk. Immunology, 19(6), 901–907. https://www.ncbi.nlm.nih.gov/pmc/articles/PMC1455671/
5. Stellflug, J., Han, D., Randel, R., & Moody, E. (1978). Plasma estrogens in the periparturient cow. Theriogenology, 10(4), 269–273. https://doi.org/10.1016/0093-691x(78)90104-8
6. Rahmany, S., & Jialal, I. (2021). Biochemistry, Chylomicron. StatPearls. https://www.ncbi.nlm.nih.gov/books/NBK545157/
7. Melnik, B.C. (2015). Milk- A Nutrient System of Mammalian Evolution Promoting mTORC1- Dependent Translation. International Journal of Molecular Sciences, 16(8), 17048-17087. doi: 10.3390/ijms160817048
8. Bhat, M. Y., Dar, T. A., & Singh, L. R. (2016). Casein Proteins: Structural and Functional Aspects. In I. Gigli (Ed.), Milk Proteins – From Structure to Biological Properties and Health Aspects. InTechOpen. https://doi.org/10.5772/60465
9. Jenness, R. (1986). Lactational Performance of Various Mammalian Species. Journal of Dairy Science, 69(3), 869–885. https://doi.org/10.3168/jds.s0022-0302(86)80478-7
10. Iverson, S. J. (2007). Milk composition and lactation strategies across mammalian taxa: implications for hand-rearing neonates. AZA Nutrition Advisory Group.
11. Urashima, T., & Messer, M. (2017). Evolution of Milk Oligosaccharides and Their Function in Monotremes and Marsupials. Evolutionary Biology: Self/Nonself Evolution, Species and Complex Traits Evolution, Methods and Concepts, 237–256. https://doi.org/10.1007/978-3-319-61569-1_13
12. Silanikove, N., Leitner, G., & Merin, U. (2015). The Interrelationships between Lactose Intolerance and the Modern Dairy Industry: Global Perspectives in Evolutional and Historical Backgrounds. Nutrients, 7(9), 7312–7331. https://doi.org/10.3390/nu7095340
13. Newman, J., Sharp, J., Enjapoori, A., Bentley, J., Nicholas, K., Adams, T., & Peat, T. (2018). Structural characterization of a novel monotreme-specific protein with antimicrobial activity from the milk of the platypus. Acta Crystallographica Section F Structural Biology Communications, 74(1), 39-45. doi: 10.1107/s2053230x17017708
14. L. (2021). Echidna [Photograph]. https://farm3.staticflickr.com/2608/4124177224_88260ac83f_o.jpg
15. Griffiths, M. (1965). Rate of growth and intake of milk in a suckling echidna. Comparative Biochemistry And Physiology, 16(4), 383-392. doi: 10.1016/0010-406x(65)90304-x
16. PÜNTENER, A., & SCHLESINGER, U. (2000). Natural Dyes. Colorants For Non-Textile Applications, 382-455. doi: 10.1016/b978-044482888-0/50040-4
17. Drescher, D., Büchner, T., McNaughton, D., & Kneipp, J. (2013). SERS reveals the specific interaction of silver and gold nanoparticles with hemoglobin and red blood cell components. Physical Chemistry Chemical Physics, 15(15), 5364. doi: 10.1039/c3cp43883j
18. Hewavisenti, R., Morris, K., O'Meally, D., Cheng, Y., Papenfuss, A., & Belov, K. (2016). The identification of immune genes in the milk transcriptome of the Tasmanian devil (Sarcophilus harrisii). Peerj, 4, e1569. doi: 10.7717/peerj.1569
19. Chapman, A. (2021). Sarcophilus harrisii (Tasmanian Devil) – captive [Photograph]. https://farm5.staticflickr.com/4047/4430046909_e92017b531_o.jpg
20. Yirka, B. (2016, October 31). Tasmanian devil milk found to have compounds with antibacterial properties. Phys.Org. https://phys.org/news/2016-10-tasmanian-devil-compounds-antibacterial-properties.html
21. De Barros, E., Gonçalves, R., Cardoso, M., Santos, N., Franco, O., & Cândido, E. (2019). Snake Venom Cathelicidins as Natural Antimicrobial Peptides. Frontiers In Pharmacology, 10. doi: 10.3389/fphar.2019.01415
22. Peel, E., Cheng, Y., Djordjevic, J., Fox, S., Sorrell, T., & Belov, K. (2016). Cathelicidins in the Tasmanian devil (Sarcophilus harrisii). Scientific Reports, 6(1). doi: 10.1038/srep35019
23. Rahilly, A. (2016, September 13). Killing superbugs with star-shaped polymers, not antibiotics. Phys.Org. https://phys.org/news/2016-09-superbugs-star-shaped-polymers-antibiotics.html
24. Carboni, D., & Tully, T. N. (2009). MARSUPIALS. Manual of Exotic Pet Practice, 299–325. https://doi.org/10.1016/b978-141600119-5.50014-7
25. Anraku, T., Fukuda, K., Saito, T., Messer, M., & Urashima, T. (2012). Chemical characterization of acidic oligosaccharides in milk of the red kangaroo (Macropus rufus). Glycoconjugate Journal, 29(2–3), 147–156. https://doi.org/10.1007/s10719-012-9372-7
26. Stannard, H. J., Miller, R. D., & Old, J. M. (2020). Marsupial and monotreme milk—a review of its nutrient and immune properties. PeerJ, 8, e9335. https://doi.org/10.7717/peerj.9335
27. Kwek, J. H., Iongh, R. D., Digby, M. R., Renfree, M. B., Nicholas, K. R., & Familari, M. (2009). Cross-fostering of the tammar wallaby (Macropus eugenii) pouch young accelerates fore-stomach maturation. Mechanisms of Development, 126(5–6), 449–463. https://doi.org/10.1016/j.mod.2009.01.003
28. Sharp, J. A., Modepalli, V., Enjapoori, A. K., Abud, H. E., Lefevre, C., & Nicholas, K. R. (2016). Milk: Milk of Monotremes and Marsupials. Reference Module in Food Science. Published. https://doi.org/10.1016/b978-0-08-100596-5.00910-0
29. Aryal, S. (2020, February 24). Carbohydrates – Monosaccharides, Disaccharides, Polysaccharides. Microbe Notes. https://microbenotes.com/carbohydrates/
30. Oligosaccharide – a carbohydrate. (n.d.). Assignmentpoint. https://www.assignmentpoint.com/science/chemistry/oligosaccharide-a-carbohydrate.html
31. Hume, I. D. (1999). Marsupial Nutrition (1st ed.). Cambridge University Press.
32. Sharma, U., & Polan, S. (2019). These animals have the fattiest, sweetest, and most potent milk in the world. Business Insider. https://www.businessinsider.com/animal-milk-fat-sugar-protein-flamingos-wallabies-aardvarks-extreme-2019-6?international=true&r=US&IR=T
33. Dasgupta, S. (2015). Seven of the Most Extreme Milks in the Animal Kingdom. Smithsonian Magazine. https://www.smithsonianmag.com/science-nature/seven-most-extreme-milks-animal-kingdom-180956588/
34. Sharp, J. A., Wanyonyi, S., Modepalli, V., Watt, A., Kuruppath, S., Hinds, L. A., Kumar, A., Abud, H. E., Lefevre, C., & Nicholas, K. R. (2017). The tammar wallaby: A marsupial model to examine the timed delivery and role of bioactives in milk. General and Comparative Endocrinology, 244, 164–177. https://doi.org/10.1016/j.ygcen.2016.08.007
35. White, J. C. D. (1953). Composition, of Whales' Milk. Nature, 171(4353), 612. https://doi.org/10.1038/171612a0
36. Ohta, K., Watarai, T., Oishi, T., Ueshiba, Y., Hirose, S., Yoshizawa, T., Akikusa, Y., Sato, M., & Okano, H. (1953). Composition of Fin Whale Milk. Proceedings of the Japan Academy, 29(7), 392–398. https://doi.org/10.2183/pjab1945.29.392
37. Fatty Acids — Overview. (n.d.). NetBiochem. https://library.med.utah.edu/NetBiochem/FattyAcids/3_1.html
38. The Editors of Encyclopaedia Britannica. (n.d.). fatty acid. Encyclopedia Britannica. https://www.britannica.com/science/fatty-acid
39. The Editors of Encyclopaedia Britannica. (n.d.). surface tension. Encyclopedia Britannica. https://www.britannica.com/science/surface-tension
40. Harding, B. (2021, January 10). How Do Whales Breastfeed Underwater? North American Nature. https://northamericannature.com/how-do-whales-breastfeed-underwater/
41. Whale Milk | Interesting Facts and Information. (2021, September 28). WHALE FACTS. https://www.whalefacts.org/whale-milk/
42. Anderson, R. R., Sadler, K. C., Knauer, M. W., Wippler, J. P., & Marshall, R. T. (1975). Composition of cottontail rabbit milk from stomachs of young and directly from gland. Journal of dairy science, 58(10), 1449–1452. https://doi.org/10.3168/jds.S0022-0302(75)84736-9
43. Maertens, L., Lebas, F., & Szendro, Z. (2010). Rabbit milk: A review of quantity, quality and non-dietary affecting factors. World Rabbit Science, 14(4), 205–230. https://doi.org/10.4995/wrs.2006.565
44. Meloni, B. P., Mastaglia, F. L., & Knuckey, N. W. (2020). Cationic Arginine-Rich Peptides (CARPs): A Novel Class of Neuroprotective Agents With a Multimodal Mechanism of Action. Frontiers in Neurology, 11. https://doi.org/10.3389/fneur.2020.00108
45. Darin, A. O., Antipova, L. V., Goz, A. R., Ippolitova, L. I., & Kumalagova, Z. H. (2021). Rabbit milk as a source of nutrition for newborns. IOP Conference Series: Earth and Environmental Science, 640(3), 032051. https://doi.org/10.1088/1755-1315/640/3/032051
46. Davies, R. R., & Rees Davies, J. A. (2003). Rabbit gastrointestinal physiology. Veterinary Clinics of North America: Exotic Animal Practice, 6(1), 139–153. https://doi.org/10.1016/s1094-9194(02)00024-5
47. Tammar wallaby. (2017, April 13). [Photograph].ZooChat https://www.zoochat.com/community/media/tammar wallaby.356542/