Courtship and Mating: The Chemistry of Pheromones and Their Evolutionary Function
Liam Fetherstonhaugh, Joseph Grotsky, Chloe Jacquet, Samuel Nidelli
Abstract
In this essay we investigate the chemistry involved in the reproductive process. Courtship and mating practices are not limited to physical and auditory pursuits. Pheromones cause innate chemical allurement between compatible animals. There are a wide range of pheromones, and each have their own specific properties and functions. Different pheromones are detected and accepted selectively ensuring mates find appropriate suitors. We provide an in-depth discussion of the four main types of pheromones: releaser, primer, signaler, and modulator. Using this knowledge, we suggest sustainable alternatives to pesticides by intervening in the courtship and mating process of insects. Additionally, we discuss the practical farming applications to increase product yield using pheromones to instigate mating. Pheromones have a wide use of applications, including hunting and protection, but in this essay, we will focus on their role in courtship and mating.
Introduction
Reproductive success is defined by a species' ability to pass their genes on to future generations. To accomplish this, they must communicate with members of the opposite sex to indicate their desire to procreate. While we are familiar with animals' complex mating rituals like birdsongs and peacock feathers, these methods span beyond just visual and aural cues. Sexual courtship practices also encompass unseen communication through chemical signals called pheromones. There are two main classes of pheromones: allomones and kairomones. Allomones increase the reproductive success of the pheromone producer whereas kairomones increase the reproductive success of the receiver. Structurally, both are usually relatively simple as they come from terpenes and fatty acids. They have very low molecular weight and can be produced by either sex. Their mechanism of production and acceptance, however, are complex and dependent on the type and structure (Regnier et al., 1968).
Production of Pheromones
Pheromones are a very broad category of compounds, and as such their source/method of synthesis can vary widely between species. Most commonly, pheromones are synthesized through enzyme aided metabolic processes in specialized glands, but in some animals, certain precursors or entire compounds can be harvested from another source, like a plant (Wyatt, 2014). Pheromones can be made up of a single compound or a mixture of many in very specific, defined ratios (Wyatt, 2014). To be classified as a pheromone, the compound or mixture need only be a consistent messenger to an entire species (Wyatt, 2014). Between individuals of the same species, the only thing that varies between their pheromone production is the amount, as different members of the same species do not produce structural variations of the same pheromone (Wyatt, 2014).
The biosynthetic pathways of sex pheromones in insects of the order Lepidoptera (butterflies and moths) are some of the most well studied due to their commercial applications in pest control. To start, there exists a neurohormone known as “pheromone biosynthesis-activating neuropeptide” (Martinez et al., 1990), which is released by endocrine glands in the brain and, as the name implies, induces biosynthesis of pheromones in pheromone glands. It is still not fully understood how this hormone achieves its function, but it is believed that it does so by regulating the supply of certain intermediates in the metabolic pathway (Martinez et al., 1990). The pheromones produced by these insects can contain a mixture of a wide variety of compounds derived from fatty acids, hydrocarbons, and isoprenoids (Fig. 1) (Blomquist et al., 2012). The precursor components required to synthesize these compounds are gained through the insect's diet, and the pheromones are then synthesized by the insect through metabolic processes in specialized tissues. The location of these pheromone producing tissues can be different for different components of the pheromone. For example, the pheromone glands located in the 9th abdominal segment of Lepidoptera have a more developed endoplasmic reticulum to aid in the metabolism of fatty acids and the production of fatty acid derived components (Blomquist et al., 2012). In contrast, hydrocarbon-based components are produced by epidermal tissue and then transported to pheromone glands to be released (Blomquist et al., 2012). The specific biosynthetic pathway involved in pheromone production varies from species to species.
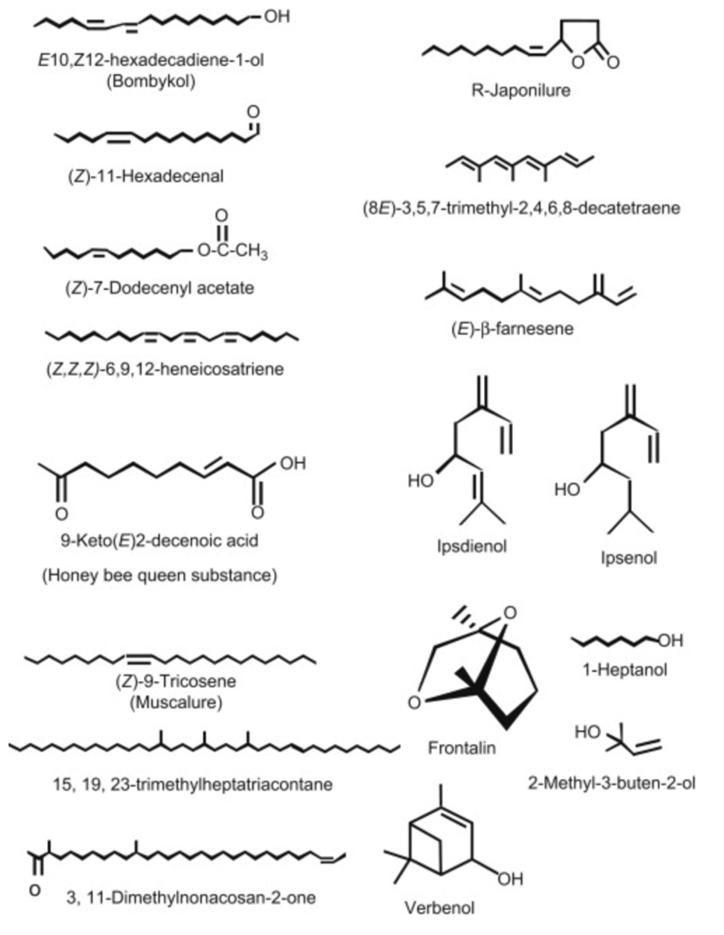
One example of a pheromone biosynthetic pathway that has been thoroughly researched is that of the species Spodoptera littoralis. This species' sex pheromone is made up of multiple components, with the main component being the fatty acid derived (Z,E)-9,11-tetradecadienyl acetate (Fig. 2). The biosynthetic pathway for this compound involves the fatty acid tetradecanoic acid being converted to (Z,E)-9,11-tetradecadienoate through the action of (E)-11 and (Z)-9 desaturating enzymes. These enzymes catalyze the creation of the double bonds in the compound one after the other through desaturation. They target specific carbons in the molecule, while selectively producing the E and Z stereoisomer, respectively. This intermediary is then reduced, and an acetyl functional group is introduced, resulting in the final product (Martinez et al., 1990). The other components of the pheromone mixture are stereoisomers of the main component, meaning they have the same chemical formula and connectivity but different orientations in space, which is a common feature of insect pheromone mixtures (Martinez et al., 1990).
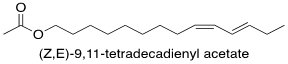
Many compounds produced by mammals have been identified as pheromones, like trimethylamine in mice and 2-methylbut-2-enal in rabbits (Wyatt, 2014), but the specific biosynthetic pathways by which these animals produce them have not been well defined. In Asian elephants, the compound frontalin is used as a pheromone, and while its synthesis in elephants has not been studied, it is thoroughly researched in the mountain pine beetle, a species that also uses frontalin as a pheromone (Wyatt, 2014). In these beetles, frontalin, an isoprenoid based pheromone also known as (1R,5R)-1,5-dimethyl-6,8-dioxa-bicyclo(3.2.1)octane, is synthesized in part through the mevalonate pathway, while the rest has been proposed by researchers but not fully defined (Fig. 3). The mevalonate pathway is a metabolic pathway that begins with acetyl-CoA and is involved in the synthesis of most isoprenoid-based pheromones (Keeling et al., 2013). In male elephants, the synthesis of frontalin occurs in a temporal gland on their face (Mori, 2010). They produce many different isomers of the compound in varying ratios depending on their maturity and hormone levels (Mori, 2010).
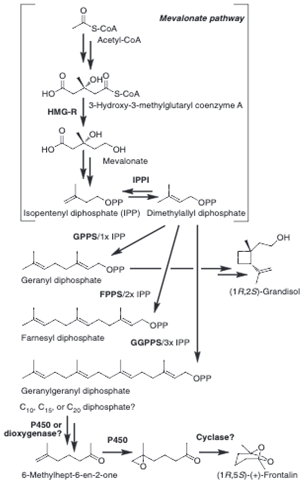
Once produced, pheromones can be released in a variety of ways, including sweat, saliva, urine, or directly released into the air. In courtship, the frequency of these processes is increased to release more pheromones for more effective mating.
Pheromone Reception and Detection
After pheromones have been produced, they are emitted to elicit a response, which can be behavioural or physiological, from a conspecific. In the vast majority of cases, the ability to recognize a pheromone and the way in which an individual responds it is something that they are born with and is consistent across the entire species, rather than being something that needs to be taught or learned (Wyatt, 2014). Understanding the response that a specific compound induces needs to be studied on a case-by-case basis, as it has been thoroughly demonstrated that the same pheromone compound can elicit a very different response in different species (Wyatt, 2014). For example, the pheromone frontalin, as mentioned in the previous section, acts as a sex pheromone in elephants while it is used to trigger aggregation in bark beetles.
Pheromones play a key role in many species courtship and mating by stimulating the optimal conditions for mating to occur. These functions can include causing the opposite sex to search for a mate, asserting dominance over others of the same sex, indicating that the individual is ready to mate, putting females into oestrus, attracting potential mates, and many other functions specific to different species mating practices (Subramoniam, 2017). In many species, pheromones are essential for communication during the execution of their complex courtship behaviours.
All organisms possess chemical senses through special chemosensory proteins that are responsible for detecting chemical signals. However, the difference in these proteins can be vast between different animals. These chemosensory proteins are used to detect pheromones primarily in the olfactory system or by gustation (taste), with very few pheromones being ingested and directly affecting the nervous system (Wyatt, 2014). Specifically in certain vertebrate species, there exists a more specialized organ called the vomeronasal organ, an accessory to the olfactory system, that can be used for pheromone detection (Wyatt, 2014).
In mammals, the primary pheromone detection system is the vomeronasal organ (Fig. 4). This organ contains many chemosensory proteins that pheromones bind to, stimulating the vomeronasal receptor neurons. This generates a signal, and the information is then sent to the accessory olfactory bulb in the brain, which is involved in olfactory processing, which then sends the signal to the medial amygdala, responsible for processing social odour signals like pheromones, which finally sends the signal to the medial hypothalamus (Fig. 5) (Brennan, 2001). The section of the hypothalamus that is sent the signal is responsible for triggering different types of genetically defined responses, thus by sending the signal to the hypothalamus the vomeronasal system can trigger both behavioural and physiological (specifically endocrine) responses to pheromone chemical signals (Brennan, 2001). This system acts similarly to and in tandem with the main olfactory system, but is more specialized, only detecting specific stimuli (Brennan, 2001).
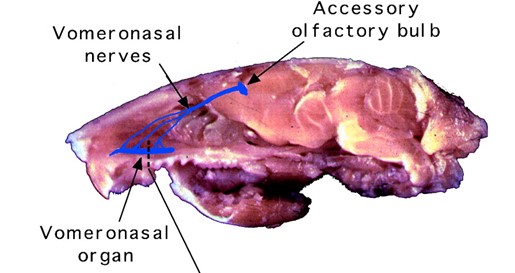
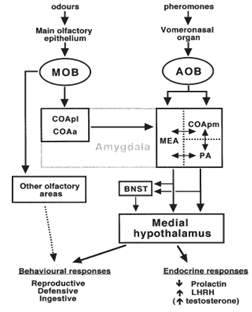
In insects, there is no vomeronasal organ. Instead, they use sensilla, which are hair-like olfactory detection systems found on the insect's antenna. Each individual sensillum will have olfactory sensory neurons (OSN) specifically for pheromone detection in addition to others used for detection of other chemical signals (Fleischer et al., 2021). To get to these sensory neurons, pheromones enter the sensilla through small pores. They then must pass through a membrane that only allows hydrophilic molecules through. Since most pheromones are hydrophobic due to being non-polar, pheromone binding proteins are produced in the sensillum to act as transport proteins to move the pheromones across the barrier to the sensory neurons (Fleischer et al., 2021). These proteins are unique in that they only bind to specific pheromones, helping to regulate which pheromones are detected. Once the pheromone reaches the OSN, they bind to chemosensory pheromone receptor proteins in the neuron's dendritic membrane, initiating a signal to be sent to the brain to induce a response (Fleischer et al., 2021). Each OSN can only detect a single component of a multi-component pheromone, and the OSNs for different components are all located on the same sensillum in certain species, while in others they can be found across many different ones (Fleischer et al., 2021). Many insect species produce pheromones that are sex-specific, i.e., one sex produces, and the other sex receives, and through evolution this results in one sex having significantly larger antennae with more developed sensilla for more effective pheromone detection (Fig. 6) (Fleischer et al., 2021).
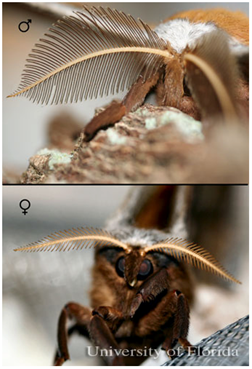
Types of Pheromones
Pheromones are chemical secretions used for communication within species of animals. They are released to alter the behavior of the receiving counterpart; that is, the accepting individual will experience a chemically induced change of emotion. While the use of pheromones is widespread throughout the animal kingdom, their specific roles vary between four primary types: releaser, primer, signaler, and modulator pheromones (Verhaeghe et al., 2013). Releaser pheromones evoke immediate behavioral effects on the receiver (Verhaeghe et al., 2013). This type of pheromone is specific to an intended response from the receiver and is used for purposes of mating, attraction, direction, distress, and identification (Jones et al., 2005). In contrast, primer pheromones take longer for a response to occur. Their release prompts a physiological series of effects by stimulating olfactory sensory neurons to signal the endocrine and neuroendocrine systems to release hormones. Over time, this affects reproductive physiology in the receiver (Verhaeghe et al., 2013). Signaler pheromones are used to supply information about the releaser; they intend to signal the animals emotional state, reproductive status, identity, recent food consumption, or genetic information (McClintock, 2009; Verhaeghe et al., 2013). This plays into courtship behaviors, whereupon receivers gauge potential mates based on their scent. Modulator pheromones adjust a receiver's mood or emotion to a set of social and physical stimuli (McClintock, 2009). They also modulate psychological reactions to scenarios without directly inducing specific behaviors (McClintock, 2009). This means that receiver behavior will not be defined by said pheromone, but instead their sensitivity to stimuli may change. Overall, while the function and role of pheromones is widespread, all types promote a method of communication between animals.
Pheromones in Boars
Pheromones are primarily sensed through olfactory receptors. Pigs have a strong reliance on smell in various behavioral contexts, and olfaction in their mating procedure is no exception (McGlone et al., 2019). Boars produce two androgen derivatives which function as sexual attractant pheromones, 5α-androst-16-en-3-one and 5α-androst-16-en-3-ol (Fig. 7) (Brennan, 2010). Both deviations are found in the concentrated saliva of boars. Generally, boars are known to salivate at high volumes, and when aroused, they salivate even more. As foam forms around the mouth accompanying the saliva, the boar shakes his head, releasing the pheromones into the surrounding air (Fig. 8). Acting as releaser pheromones, 5α-androst-16-en-3-one and 5α-androst-16-en-3-ol target estrous sows (Brennan, 2010). In response, these sows are inclined to a receptive mating stance to permit mounting by the boar (Brennan, 2010; Dorries et al., n.d.). Resultantly, mating occurs, premediated by the androgen pheromone release.
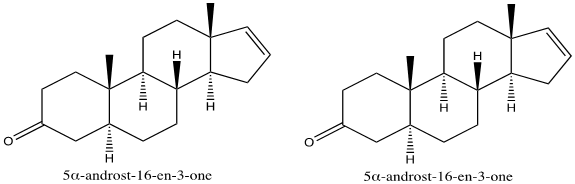
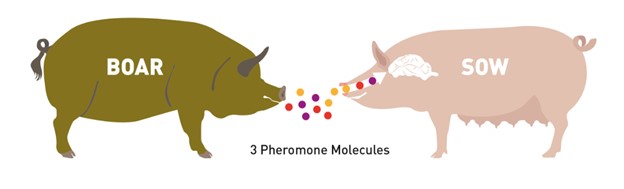
The features of boar androgen pheromones have found application in pig farms. As the natural hormone is both fast and effective, engineers and chemists have synthesized androsterone into a product known as BOARMATE (Wysocki et al., 2004). BOARMATE is used by farmers to solicit artificial insemination. By releasing a concentrated version of the pheromone into surrounding air of receptive sows, mating procedures are conducted on demand. Ethics aside, this demonstrates the capabilities of pheromones themselves and prominently, the potentials of engineering and chemistry.
Pheromones in Elephants and Relation with Moths
Asian elephants, Elephas maximus, deliver pheromones in a unique process to induce mating. Several weeks prior to ovulation, female elephants secrete pheromones in their urine to evoke non-habituating responses by the male counterpart, primarily flehmens response (Rasmussen et al., 1997). Flehmens response consists of the male elephant positioning its trunk near the female's urine and subsequently lifting the tip to the roof of its mouth. This leads to the vomeronasal organ, an important component of pheromone reception (Fig. 9). The high frequency of this action over an extended period, alongside a series of smaller induced responses, supports male sexual arousal behavior (Rasmussen et al., 1997). As a result, the male experiences erections and penile searching motions, which may be accompanied by mounting and copulation if a female is available (Rasmussen et al., 1997). The female-to-male sex pheromone responsible for said behavior is (Z)-7-dodecenyl acetate (Fig. 10) (Rasmussen et al., 1997). This is a both a primer and signaler pheromone eliciting a chain of prolonged reactions, rather than immediate effects.
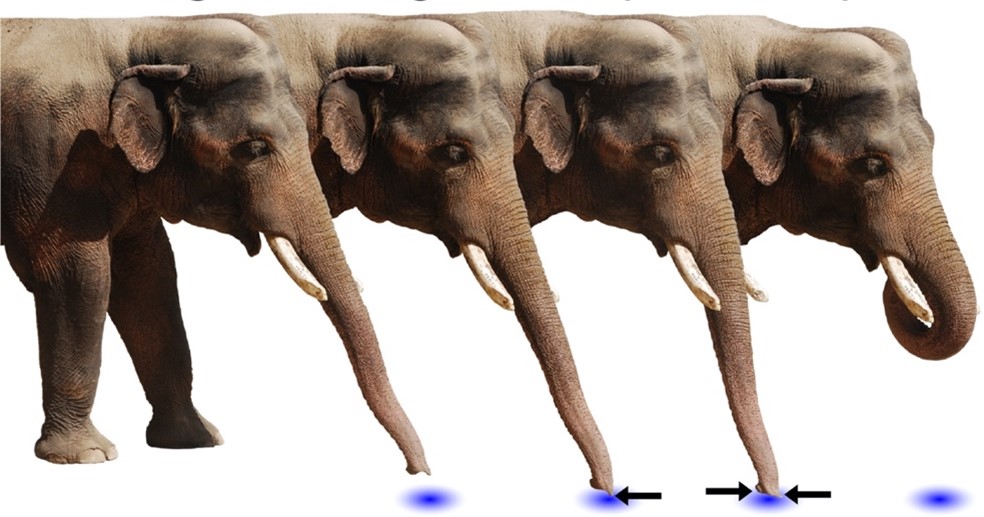
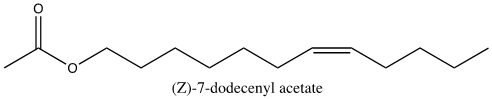
Interestingly, elephant pheromones are not entirely unique. Upon comparison, both elephants and some 140 species of moth utilize (Z)-7-dodecenyl acetate as a sex pheromone (Fig. 11) (Wyatt, 2003). It is important to note however that despite using the same pheromone, it is extremely unlikely for confusion to occur between the vastly different species. Most notably, moth pheromones encompass multiple components – often five or six – to the elephant's one (Wyatt, 2003). As a result, the respective males for each species would have a difficult challenge discerning the similarity. Another key point is that elephants do not possess the sensory system of a moth, so it is unlikely that any elephant would be able to pick up the relatively minute quantities released by a female moth (picograms per hour) (Wyatt, 2003). And, most obviously, neither species would be compatible should arousal stimulation occur. The compelling similarity in the chemical makeup of sexual pheromones does present an interesting connection to the common origin of life. That is, basic enzyme pathways are frequent amongst multicellular organisms, with molecules being shared throughout vastly different animals (Wyatt, 2003).
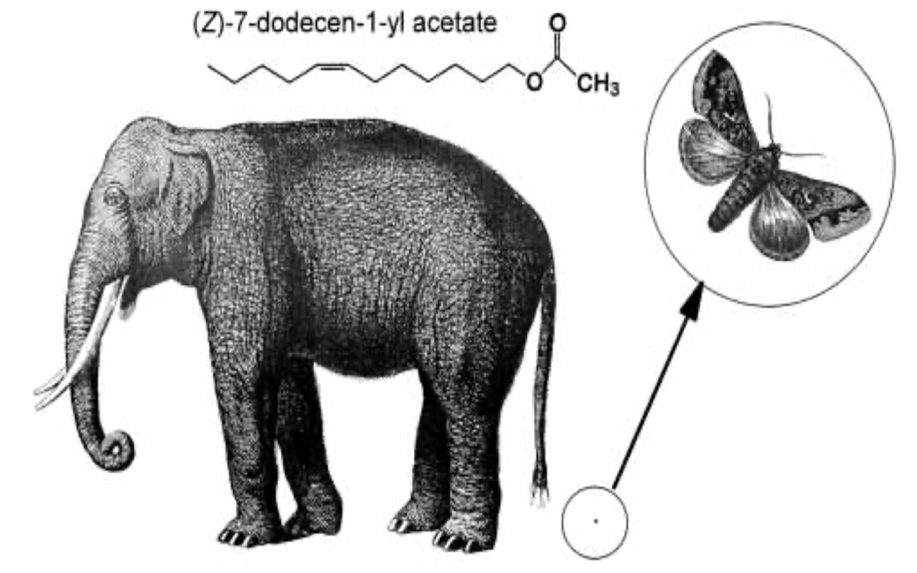
Bombykol: Releaser Sex Pheromone within Bombyx Mori
Within courtship and mating, a very well-documented case of a releaser pheromone that has the role of a sex pheromone is the Bombykol pheromone within the Bombyx Mori, a type of silkworm moth (Regnier et al., 1968). The pheromone (Bombykol) was discovered in 1959 by Butenandt et al (Regnier et al., 1968). It is released into the air by the female moth through turning their abdominal sacs inside out, immediately after exiting the cocoon. Once released, the male Bombyx Mori will receive the pheromone through the BmOR1 odorant receptor neuron, which is found in the antennal trichoid sensilla (Syed et al., 2010). After reception, the male Bombyx Mori immediately develops an attraction to the females, which is shown by fluttering of the wings (Fig. 12) (Regnier et al., 1968).
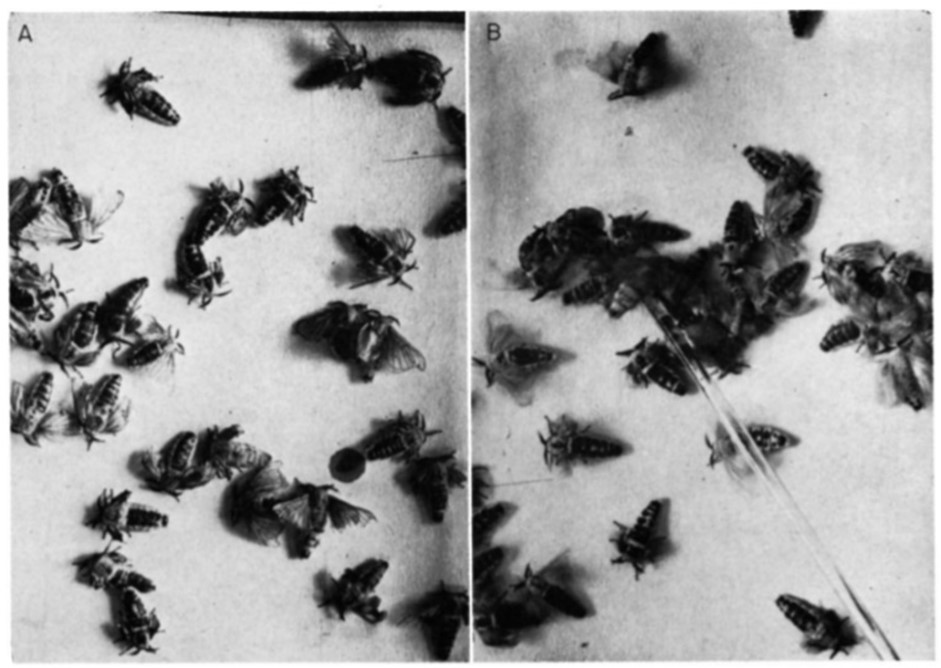
IR spectrometry was used by Butenandt et al. to identify the chemical makeup of the pheromone (Regnier et al., 1968). IR spectrometry is a tool used to help find a compound's chemical makeup by analysing its interaction with infrared light, and it plots measured IR intensity vs the frequency of light (Chemistry LibreTexts, 2020a). This study can be conducted because chemical bonds will vibrate when they absorb infrared light (Chemistry LibreTexts, 2020a). Each molecule's vibrations can be broken down into constituents, which is what shows the chemical makeup since each constituent vibrates at a specific frequency (Chemistry LibreTexts, 2020a). By looking at what frequencies interact the most with the molecule, you can determine what constituents are present. This method of analysis showed that the pheromone contained an alcohol group (-OH) since a peak in the IR spectrum was 3320cm (Pherobase). Additionally, it was shown through IR spectrometry that the compound contained a conjugated double bond in the cis-trans arrangement (Regnier et al., 1968). To find the parent chain, hydrogenation was used, which is a process that will saturate organic compounds (i.e., take away their double bonds) by reacting H2 in the presence of a catalyst such as nickel, platinum or palladium (Lew, 2020). When conducting this process, it was shown that the product was cetyl alcohol, meaning that the parent chain was a 16-carbon chain (Regnier et al., 1968). To locate where the double bonds were situated, oxidative cleavage of Bombykol with the presence of KMnO4 was conducted (Regnier et al., 1968). Oxidative cleavage in the presence of KMnO4 will form a mixture of carboxylic acids and ketones where the double bonds once where (“Oxidative Cleavage [KMnO4],” n.d.). So, given the previous information, the final chemical makeup of the pheromone is 10,12-hexadecadien-1-ol (Fig. 13).

The rate of effusion of the molecule is given by the formula (Chemistry LibreTexts, 2020c):
Rate \space of \space effusion = \frac{1}{\sqrt{molar \space mass}}
The rate of effusion of a molecule corresponds to the speed at which they will travel (Chemistry LibreTexts, 2020c). Molecules with a lower rate of effusion will travel slower compared to molecules with a higher rate of effusion. Given that the molar mass of 10,12-hexadecadien-1-ol is 238.41 g/mol (National Center for Biotechnology Information, 2021b), the rate of effusion is around 0.0647.
Finally, the intermolecular forces of Bombykol were analyzed. Intermolecular forces are the interactive forces that reside between molecules based on the particles around them, here are the four types: London Dispersion Forces, Dipole-Dipole, Hydrogen bonding, and Ion-Dipole (Chemistry LibreTexts, 2020b). The intermolecular forces found for this compound is primarily hydrogen-bonding, since the terminal -OH group has a positive pole in the H and a negative pole in the O (since O is quite electronegative).
Queen Mandibular Pheromone: Primer Sex Pheromone within Apis Mellifera
The Queen Mandibular Pheromone (QMP) pheromone (E)-9-oxodec-2-enoic-acid (9-ODA) (Fig. 14) plays a major role in the honeybee (Apis mellifera) society and is one of the most well documented pheromones within the species (Bortolotti & Costa, 2014). The pheromone is released by the queen mandibular gland, which are located near the base of the mandible, inside the head of the queen bee. The major use of the (E)-9-oxodec-2-enoic-acid pheromone is its role in producing the “queen signal” (Bortolotti & Costa, 2014). The “queen signal” is a complex mixture of primer pheromones, including (E)-9-oxodec-2-enoic-acid, which makes it so that the worker bees have behavioral and physiological modifications to maintain homeostasis within the colony (Bortolotti & Costa, 2014). Within a honeybee's colony, homeostasis is defined as the maintenance of the hierarchy, where the queen reigns supreme and has all the reproductive power (Bortolotti & Costa, 2014). The “queens signal” affects the worker bees to stimulate them to engage in activities to help the colony such as building and foraging.It also inhibits the workers from reproducing (Bortolotti & Costa, 2014). Fan et al. (2010) conducted a study which showed how the Queen Mandibular Pheromone will alter the cuticular hydrocarbon patterns in the worker bees of the colony (Illustrated Glossary of Organic Chemistry, n.d.). Cuticular hydrocarbon patterns are cues that help worker bees distinguish which bees are intruders and which are a part of their colonies (Fan et al., 2010).
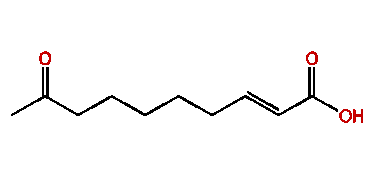
Butler et al. (1962) conducted an experiment trying to isolate the QMP, during this experiment, the IR spectrum (Fig. 15) of (E)-9-oxodec-2-enoic-acid was used to properly identify all the components within the molecule. The use and explanation of IR spectroscopy was done in the previous example. The broad bandwidth at 2700 to 2540cm-1 shows that the molecule contains a carboxylic acid (Fan et al., 2010). Unconjugated and conjugated carbonyl groups were shown by the bands of 1705cm-1 and 1695cm-1 to 1690cm-1 (Bulter et al., 1962). The 995cm-1 indicated a trans configuration of a double bond (Bulter et al., 1962), the band at 1380 to 1375cm-1 indicates that a methyl group is terminal (Bulter et al., 1962), and the band at 1320cm-1 shows that there is ketone group within the molecule (Bulter et al., 1962). The absence of a band at 720cm-1 shows that this molecule is a long chain (Bulter et al., 1962). To identify where the ketone group is Butler et al. used a variety of techniques. One of the techniques used was to run an iodoform reaction with the isolated QMP and compare the chromatography with the non-trans-2-enedoic acid. The iodoform reaction is done by oxidizing methyl ketones with OH- (aq) and I2 to form carboxylate (Illustrated Glossary of Organic Chemistry, n.d.). The result showed a completed iodoform reaction and a direct correlation with the chromatography of non-trans-2-enedoic acid. The rate of effusion of QMP where its molar mass is 184.23g/mol (National Center for Biotechnology Information, 2021a) is around 0.0736. This rate of effusion means that the molecule will travel faster through the air compared to the previous example (Bombykol), since it's rate of effusion is higher. The primary intermolecular forces found in this compound, similarly to the previous example, is hydrogen-bonding, since the terminal -OH group has a positive pole in the H and a negative pole in the O (since O is quite electronegative).
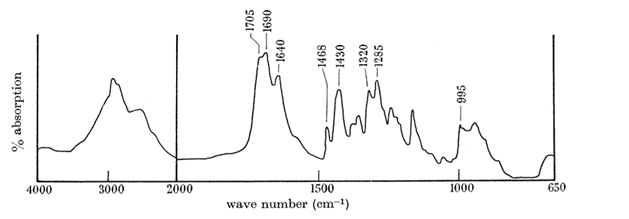
Engineering Applications
The properties of pheromones can be applied to control pest populations and reduce the utilization of harmful chemicals. With the daunting effects of climate change, sustainable innovation is of pressing concern. Pesticides are widely used worldwide with around 4.7 billion pounds of pollutants used in 1995. As time has passed these numbers have only risen. To consider omitting pesticides altogether places the burden onto farmers and is not realistic long term. Many efforts are focused on prioritizing less harmful chemicals; however, an optimal resolution would omit synthetic chemicals altogether (Tabata, 2015). Pheromone disruption provides a sustainable alternative without harming the livelihoods of small farms.
When female calling pheromones are imitated, they can be used to distract males of the species, hindering them from finding real mates. When a female is ready to engage in mating, she releases a flume of pheromones. The male is then able to easily locate her for procreation. However, if multiple pheromone imitations are placed throughout the habitat (Fig. 16), it becomes impossible for him to distinguish between the synthetic dispensers and the real females (Fig. 17). This process is called pheromone trapping. Therefore, mating disruption is an effective strategy for species where the mating ritual includes calling pheromones to locate mates (Brunner, 1993).
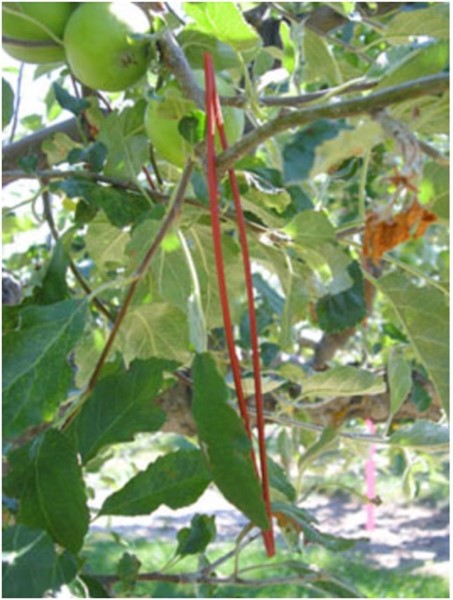
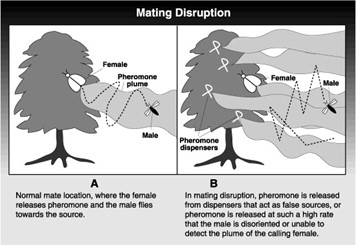
Specificity of the pheromones is highly important to the effectiveness of the disruption. A mathematical model published by the International Conference on Mathematics, Statistics, and Data Science showed the population growth relative to the effectiveness of the control, where the control is defined by the number of females which are concealed using pheromone traps. As seen in figure 18, the efficacy of the control had a strong relationship with the populations of larva, males, and unfertilized vs fertilized females. Even between 90 and 100 percent control effectiveness, there is a huge gap in the larva production after around 4 days. Therefore, successful implementation requires a specialized control. This allows farmers to reduce costs and while maximizing efficacy (Fitri et al., 2021).
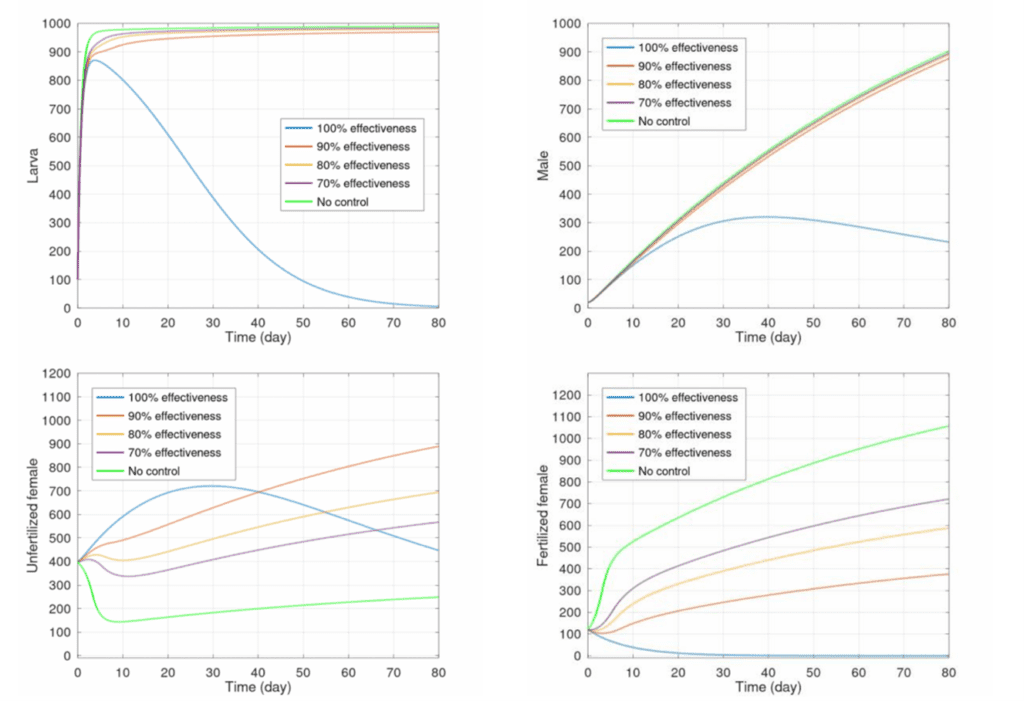
Mealy bugs are an example of successful pest control using mating disruption (Tabata, 2015). Lavandulol (2-isopropenyl-5-methyl-4-hexen-1-ol), found in lavender, is characterized by different connections than other chemicals in its family. This makes it easily distinguishable from other monoterpenes. This same unique linkage is found in the pheromones of mealybugs making lavender an effective specific control for the process of mating disruption. By using lavandulol as a base, the mating pheromone isolavandylyl butyrate can be synthesized. A field study by Tabata (2015) found this purification was highly effective at reducing the population of Japanese Mealybugs. Even without the purification of lavandulol, populations were diminished. Figure 19 shows the number of males attracted to the synthetic pheromone traps per day during the study. It can be observed that there is statistically significant increase in males attracted to the pure attractant than the lavender attractant, with a p-value of less than 0.05. However, as seen in figure 20, the rate of reproduction between the two were very similar and both highly effective comparatively to the control. A possible explanation for this is lowered receptive sensitivity caused by the lavender. Even males that were not attracted to the traps are affected by the introduction of the chemical. The large amounts present in the air desensitize the males to the real mating pheromones. Thus, they are unable to detect with such accuracy the pheromones using their antenna and central nervous system. Therefore, the complicated and expensive process of purifying the lavender is unnecessary and mating disruption becomes an inexpensive and sustainable alternative to traditional pest control techniques (Tabata, 2015).
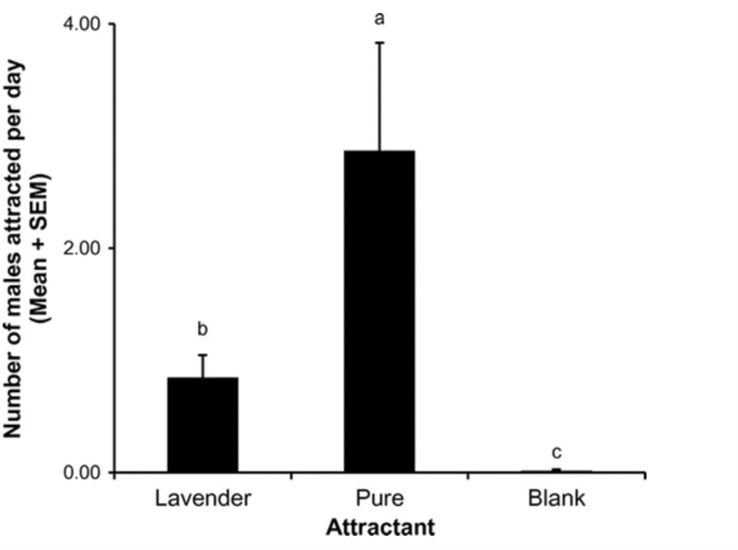
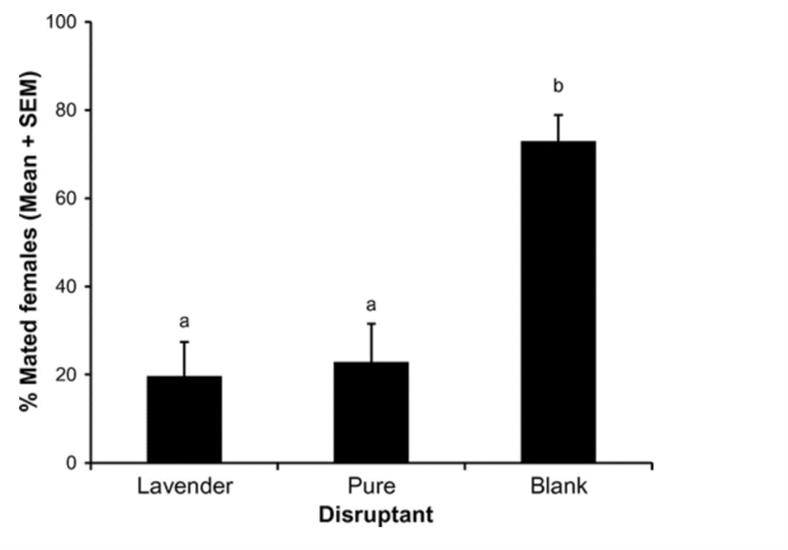
Conclusion
In this paper, we discussed the chemistry behind pheromone production. The biosynthesis process is primarily done in specialized glands, which can either be endocrine or exocrine. The reception of pheromones is distinct for mammals and insects. Mammals primarily use the vomeronasal organ to detect pheromones. In contrast, insects lack the vomeronasal organ, so, instead, they utilize sensilla. Pheromones play a large role within courtship and mating because they offer physiological and behavioral changes within a species. These changes can improve the homeostasis of a colony, such as the QMP pheromone in the queen honeybee. Multiple examples of sex pheromones were shown such as the two androgen derivatives of boars, which is released by the male and received by the female who will position themselves into a mating stance, the urinary pheromone in female elephants which elicits a series of non-habituating responses by the male which over time increases sexual arousal, and the Bombykol pheromone in commercial silkworm moths which is released by the female and will induce sexual attraction within males. These complex compounds have and continue to inspire humans to utilize pheromones in the natural world, such as their role in pest control, which minimizes the use of harsh chemicals.
References
Bestmann, H. J., Süß, J., & Vostrowsky, O. (1981). Pheromone XXXIV. Synthese konjugiert‐ungesättigter Lepidopterenpheromone und Analoga. Liebigs Annalen der Chemie, 1981(12), 2117-2138.
Blomquist, G. J., Jurenka, R., Schal, C., & Tittiger, C. (2012). 12 – Pheromone Production: Biochemistry and Molecular Biology. In L. I. Gilbert (Ed.), Insect Endocrinology (pp. 523-567). Academic Press. https://doi.org/https://doi.org/10.1016/B978-0-12-384749-2.10012-3
Bortolotti, L., & Costa, C. (2014). Frontiers in Neuroscience Chemical Communication in the Honey Bee Society. In C. Mucignat-Caretta (Ed.), Neurobiology of Chemical Communication. CRC Press/Taylor & Francis © 2014 by Taylor & Francis Group, LLC.
Brennan, P. A. (2001). The vomeronasal system. Cell Mol Life Sci, 58(4), 546-555. https://doi.org/10.1007/PL00000880
Brennan, P. A. (2010). Pheromones and Mammalian Behavior. In A. Menini (Ed.), The Neurobiology of Olfaction. https://www.ncbi.nlm.nih.gov/pubmed/21882427
Brunner, J. F., & Knight, A. (1993). Mating Disruption. Washington State University. http://treefruit.wsu.edu/crop-protection/opm/mating-disruption/
Butler, C. G., Callow, R. K., & Johnston, N. C. (1962). The isolation and synthesis of queen substance, 9-oxodec-trans-2-enoic acid, a honeybee pheromone. Proceedings of the Royal Society of London. Series B. Biological Sciences, 155(960), 417-432. https://doi.org/doi:10.1098/rspb.1962.0009
Chemistry LibreTexts. (2020a, August 21). Infrared: Interpretation. LibreTexts. Retrieved November 4, 2021, from https://chem.libretexts.org/Bookshelves/Physical_and_Theoretical_Chemistry_Textbook_Maps/Supplemental_Modules_(Physical_and_Theoretical_Chemistry)/Spectroscopy/Vibrational_Spectroscopy/Infrared_Spectroscopy/Infrared:_Interpretation
Chemistry LibreTexts. (2020b, August 23). 12.6: Types of Intermolecular Forces- Dispersion, Dipole–Dipole, Hydrogen Bonding, and Ion-Dipole. LibreTexts. Retrieved November 4, 2021, from https://chem.libretexts.org/Courses/Sacramento_City_College/SCC:_CHEM_300_-_Beginning_Chemistry/SCC:_CHEM_300_-_Beginning_Chemistry_(Alviar-Agnew)/12:_Liquids_Solids_and_Intermolecular_Forces/12.06:_Types_of_Intermolecular_Forces-_Dispersion,_Dipole–Dipole,_Hydrogen_Bonding,_and_Ion-Dipole
Chemistry LibreTexts. (2020c, September 1). 2.9: Graham's Laws of Diffusion and Effusion. LibreTexts. Retrieved November 4, 2021, from https://chem.libretexts.org/Bookshelves/Physical_and_Theoretical_Chemistry_Textbook_Maps/Map:_Physical_Chemistry_for_the_Biosciences_(Chang)/02:_Properties_of_Gases/2.9:_Graham's_Laws_of_Diffusion_and_Effusion
Compound – E2-9-oxo-10Acid. (n.d.). The Pherobase. Retrieved November 5, 2021, from https://www.pherobase.com/database/synthesis/synthesis-detail-E2-9-oxo-10Acid.php
Dorries, K. M., Adkins-Regan, E., & Halpern, B. P. (1997). Sensitivity and behavioral responses to the pheromone androstenone are not mediated by the vomeronasal organ in domestic pigs. Brain Behav Evol, 49(1), 53-62. https://doi.org/10.1159/000112981
Fan, Y., Richard, F.-J., Rouf, N., & Grozinger, C. (2010). Effects of queen mandibular pheromone on nestmate recognition in worker honeybees, Apis mellifera. Animal Behaviour, 79, 649-656. https://doi.org/10.1016/j.anbehav.2009.12.013
Fitri, I. R., Bakhtiar, T., Hanum, F., & Kusnanto, A. (2021). The Characteristics and Optimal Implementation of Mating Disruption in Managing Pest Insect Population. Journal of Physics: Conference Series, 1863(1), 012001. https://doi.org/10.1088/1742-6596/1863/1/012001
Fleischer, J., & Krieger, J. (2021). 12 – Molecular mechanisms of pheromone detection. In G. J. Blomquist & R. G. Vogt (Eds.), Insect Pheromone Biochemistry and Molecular Biology (Second Edition) (pp. 355-413). Academic Press. https://doi.org/https://doi.org/10.1016/B978-0-12-819628-1.00012-2
Illustrated Glossary of Organic Chemistry. (n.d.). University of California, Los Angeles. Retrieved November 5, 2021, from https://www.chem.ucla.edu/~harding/IGOC/I/iodoform_reaction.html
Jones, G. R., & Parker, J. E. (2005). PHEROMONES. In P. Worsfold, A. Townshend, & C. Poole (Eds.), Encyclopedia of Analytical Science (Second Edition) (pp. 140-149). Elsevier. https://doi.org/https://doi.org/10.1016/B0-12-369397-7/00733-0
Keeling, C. I., Chiu, C. C., Aw, T., Li, M., Henderson, H., Tittiger, C., Weng, H. B., Blomquist, G. J., & Bohlmann, J. (2013). Frontalin pheromone biosynthesis in the mountain pine beetle, Dendroctonus ponderosae, and the role of isoprenyl diphosphate synthases. Proc Natl Acad Sci U S A, 110(47), 18838-18843. https://doi.org/10.1073/pnas.1316498110
Keverne, E. B. (1999). The vomeronasal organ. Science, 286(5440), 716-720. https://doi.org/10.1126/science.286.5440.716
LaDue, C. A., Goodwin, T. E., & Schulte, B. A. (2018). Concentration-dependent chemosensory responses towards pheromones are influenced by receiver attributes in Asian elephants. Ethology, 124(6), 387-399. https://doi.org/https://doi.org/10.1111/eth.12741
Levitan, L. (2000). “How to” and “why”:: assessing the enviro–social impacts of pesticides. Crop Protection, 19(8), 629-636. https://doi.org/https://doi.org/10.1016/S0261-2194(00)00083-1
Lew, J. (2020, September 13). Catalytic Hydrogenation of Alkenes. LibreTexts. Retrieved November 5, 2021, from https://chem.libretexts.org/Bookshelves/Organic_Chemistry/Supplemental_Modules_(Organic_Chemistry)/Alkenes/Reactivity_of_Alkenes/Catalytic_Hydrogenation
Martinez, T., Fabrias, G., & Camps, F. (1990). Sex pheromone biosynthetic pathway in Spodoptera littoralis and its activation by a neurohormone. J Biol Chem, 265(3), 1381-1387. https://www.ncbi.nlm.nih.gov/pubmed/2295634
McClintock, M. K. (2009). Pheromones in Humans and Social Chemosignals. In L. R. Squire (Ed.), Encyclopedia of Neuroscience (pp. 617-623). Academic Press. https://doi.org/https://doi.org/10.1016/B978-008045046-9.01707-1
McGlone, J. J., Garcia, A., & Rakhshandeh, A. (2019). Multi-Farm Analyses Indicate a Novel Boar Pheromone Improves Sow Reproductive Performance. Animals (Basel), 9(2). https://doi.org/10.3390/ani9020037
Mori, K. (2010). 4.01 – Overview and Introduction. In H.-W. Liu & L. Mander (Eds.), Comprehensive Natural Products II (pp. 1-7). Elsevier. https://doi.org/https://doi.org/10.1016/B978-008045382-8.00736-X
National Center for Biotechnology Information. (2021a). PubChem Compound Summary for CID 1713086, Queen substance. Retrieved November 5, 2021, from https://pubchem.ncbi.nlm.nih.gov/compound/Queen-substance
National Center for Biotechnology Information. (2021b). PubChem Compound Summary for CID 5353007, 10,12-Hexadecadien-1-ol. Retrieved November 4, 2021, from https://pubchem.ncbi.nlm.nih.gov/compound/10_12-Hexadecadien-1-ol
Oxidative Cleavage [KMnO4]. (n.d.). ChemistryScore. Retrieved November 4, 2021, from https://chemistryscore.com/oxidative-cleavage-kmno4/
Rasmussen, L. E. L., Lee, T. D., Zhang, A., Roelofs, W. L., & Daves, G. D. (1997). Purification, Identification, Concentration and Bioactivity of (Z)-7-Dodecen-1-yl Acetate: Sex Pheromone of the Female Asian Elephant, Elephas maximus. Chemical Senses, 22(4), 417-437. https://doi.org/10.1093/chemse/22.4.417
Regnier, F. E., & Law, J. H. (1968). Insect pheromones. J Lipid Res, 9(5), 541-551. https://www.ncbi.nlm.nih.gov/pubmed/4882034
Subramoniam, T. (2017). Chapter 6 – Sex Pheromones. In T. Subramoniam (Ed.), Sexual Biology and Reproduction in Crustaceans (pp. 159-173). Academic Press. https://doi.org/https://doi.org/10.1016/B978-0-12-809337-5.00006-X
Syed, Z., Kopp, A., Kimbrell, D. A., & Leal, W. S. (2010). Bombykol receptors in the silkworm moth and the fruit fly. Proc Natl Acad Sci U S A, 107(20), 9436-9439. https://doi.org/10.1073/pnas.1003881107
Tabata, J., Teshiba, M., Shimizu, N., & Sugie, H. (2015). Mealybug mating disruption by a sex pheromone derived from lavender essential oil. Journal of Essential Oil Research, 27(3), 232-237. https://doi.org/10.1080/10412905.2015.1007219
The Importance of Odour. (n.d.). Boar Better. Retrieved November 4, 2021, from https://www.boarbetter.com/en/the-importance-of-odour/
Verhaeghe, J., Gheysen, R., & Enzlin, P. (2013). Pheromones and their effect on women's mood and sexuality. Facts Views Vis Obgyn, 5(3), 189-195. https://www.ncbi.nlm.nih.gov/pubmed/24753944
Wyatt, T. (2003). Animals in a chemical world. In Pheromones and Animal Behaviour: Communication by Smell and Taste (pp. 1-22). Cambridge University Press. https://doi.org/DOI: 10.1017/CBO9780511615061.002
Wyatt, T. (2014). Pheromones and animal behavior: Chemical signals and signatures, second edition. https://doi.org/10.1017/CBO9781139030748
Wysocki, C. J., & Preti, G. (2004). Facts, fallacies, fears, and frustrations with human pheromones. Anat Rec A Discov Mol Cell Evol Biol, 281(1), 1201-1211. https://doi.org/10.1002/ar.a.20125