A Taste of Trunks: Self-Cleaning Properties and Gustatory Responses of the Proboscis
Han Ru Liu, Letizia Marello, Liam Sterne, Kori Zhang
Abstract
The trunk, or proboscis, is an important organ for many organisms, especially insects dependent on fluid-feeding, such as butterflies, mosquitoes, and bees. The structure of the trunk allows the organism to take up liquid food, probe soil, etc., while remaining clean thanks to certain hydrophobic/hydrophilic properties of the organ. This paper explores the relationship between the structure and wetting characteristics of the proboscis and its self-cleaning properties. It further delves into the gustatory response of insect chemosensillia and the motivations behind the proboscis extension response. The proboscis has interesting components that allow the organ to precipitate a response when in contact with certain foods, developing habitual neurological behavior. The neural projections from the antenna and proboscis gustatory sensory neurons are transmitted to mechanical response as a proboscis extension response. Furthermore, the taste preferences towards sugars, alcohols and amino acids of butterfly species, musk shrews and elephants will be explored. Finally, the sensory organs on the proboscis used to detect chemical signals and cues will be examined in female mosquitoes as they are heavily reliant on these sensory organs to detect carbon dioxide, lactic acid, and volatile fatty acids present on a possible host for the protein content in its blood.
Keywords: trunk, proboscis, hydrophobic/hydrophilic properties, gustatory response, chemosensory sensilla, sensory organs
Introduction
Over time, environmental changes and genetic adaptations have allowed different species to develop a diet appropriate for their survival. Some animals feed on fresh fruit and woody plants, while others rely on animal meat. Some organisms consume algae, while others can live off of decomposed organic matter. For most insects, their diet depends on the proper function of their proboscis, an elongated feeding tube that takes up liquid food like nectar and phloem. An important aspect that enables the butterfly proboscis to work correctly is its self-cleaning properties. The structure and wetting characteristics — the degree of hydrophilicity of the proboscis — are strictly related to its self-cleaning abilities. The organ is divided into two parts: a hydrophobic region that repels water and a hydrophilic part that attracts water. This dichotomy prevents any contamination by debris or bacteria in the proboscis, allowing the organ to maintain its functionalities. Foraging and feeding are vital characteristics of insect proboscis and its interactions with our complex chemical environment are key determinants of its behavior. Both olfactory and gustatory responses are necessary mannerisms to ensure efficient and effective feeding. While gustatory preference discloses incredible co-evolution between animals and plants, neurological olfactory activity has fascinating impacts on feeding mechanisms and activity in both butterflies and mosquitoes.
Butterfly proboscis
Self-cleaning properties of the proboscis
The proboscis is an essential organ for fluid-feeding insects like the Lepidoptera butterfly that uses it to feed. The proboscis consists of two C-shaped fibers, the galae, that are joined together and form the food canal of the proboscis (Fig. 1). The two are linked by rows of overlapping, fence-like dorsal and ventral legulae (Pometto, 2019).
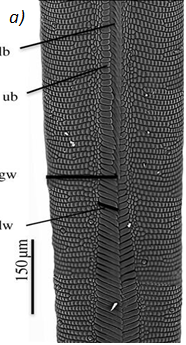
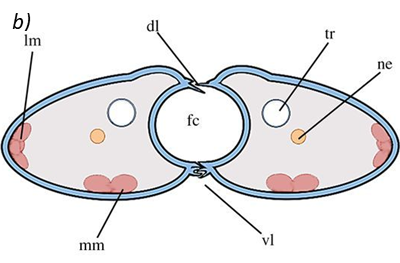
Fig. 1 (a) Proboscis of Danaus Plexippus where the upper (ub) and lower (lb) branch of the legula (lw) and the galea (gw) are shown. (b) Schematic of a cross section of the proboscis showing two C-shaped galeae with lateral and median intrinsic muscles (lm and mm), nerves (ne) and tracheae (tr). The galeae are held together by overlapping dorsal legulae (dl) and interlinking ventral legulae (vl), forming a food canal (fc) (Lehnert et al., 2013).
The food canal was first approximated to be a circular cylinder tube, following the drinking straw model, but it has been discovered that the proboscis is far from a straw. It can be better represented with a conical shape since the proboscis tapers outwards: both the thickness and width increase gradually from the tip to the base of the proboscis (Fig. 2).
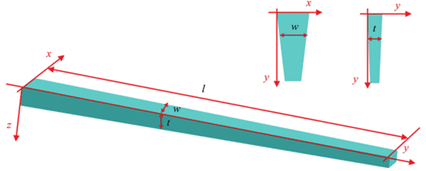
Fig. 2 Modeled conical structure of a tapered proboscis where (w) is width, (t) thickness and (l) is length (Chen et al., 2020).
Lepidoptera, as many insects, can feed from pools and films of liquid as well as porous substrates such as wet soil, or rotting fruits. Therefore, an interesting question arises: how does the proboscis collect nectar and probe soil while remaining clean and free of contamination of debris and bacteria?
Experimental analyses revealed that the proboscis has a curious and maybe counterintuitive property: it is composed of a hydrophilic drinking region which represents 5-17 % of the total proboscis area (depending on the species), where water is attracted to the surface; and a hydrophobic non-drinking region where water is repelled from the surface (Lehnert et al., 2013) (Figure 3). The drinking region refers to the tip of the proboscis, which is hydrophilic to assist feeding, while the rest of the surface is hydrophobic, crontibuting to self-cleaning. Hydrophobicity is useful to prevent adherence of substances like dirt, debris, bacterias. Even though hydrophobic, the non-drinking region is still capable of fluid uptake thanks to the hydrophilicity of the dorsal legulae (Pometto, 2019). The legulae are hydrophilic, which helps fluid uptake,channeling it towards the food canal, as well as chemosensory proteins, present in the proboscis to reduce the pressure involved in sucking.
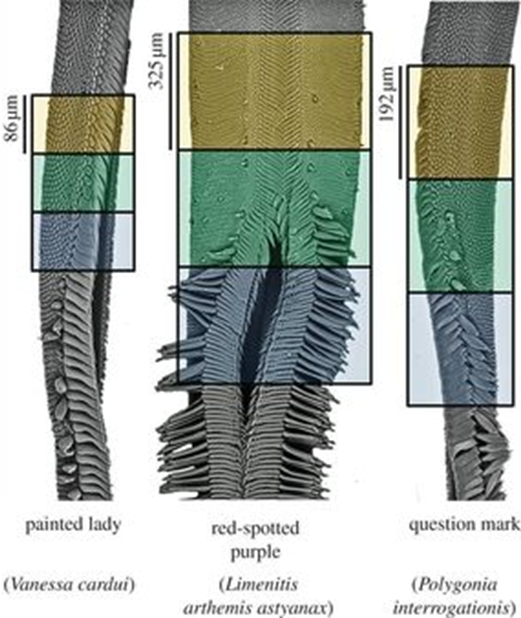
Fig. 3 Scanning electron micrographs of butterfly proboscises and associated wettability regions. The upper (orange) part represents the hydrophobic region, the middle (green) represents the transition and the lower (blue) the hydrophilic region. A single galea is shown for V. cardui and P. interrogationis; both galeae are shown for other species (Lehnert et al., 2013).
The hydrophobicity of the non-drinking part is caused by the hydrophobic chitin and different types of proteins, lipids and waxes (esters of long-chain alcohols and fatty acids). This is explained by the bonds between atoms that are found in those molecules and structures. Those bonds have the particularity of being non-polar, like the bond between the hydrogen and carbon atoms in lipids. The entire molecule has a neutral charge which is the opposite of water where the bonds between two hydrogen atoms and one oxygen causes the molecule to have a slight negative charge on one side and a slight positive charge on the other. Water is, therefore, polar and polar molecules are repelled by non-polar ones explaining hydrophobicity.
The wetting of a surface (its hydrophilicity) is measured by the contact angle formed between the surface and a droplet of a liquid (Figure 4). For water droplets the contact angle is smaller than 90° for hydrophilic surfaces and larger than 90° for hydrophobic surfaces. Chitinous and waxed surfaces indeed have high contact angles with water droplets, a feature of hydrophobic surfaces (picture) thatfacilitates cleaning since it prevents fluids from wetting them (Krenn et al., 2019).
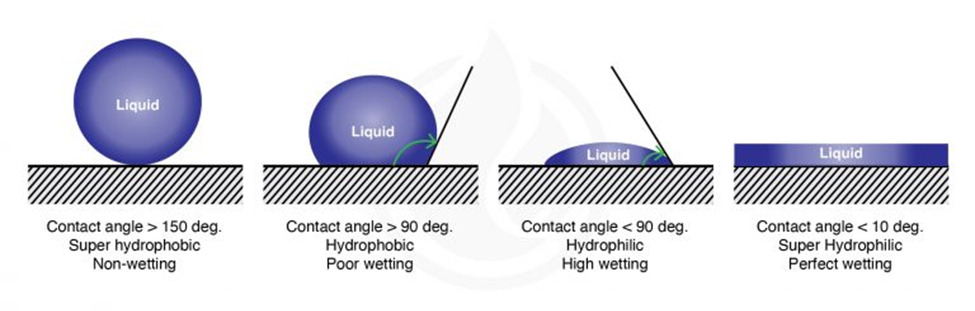
Fig. 4 Water contact angle of different types of surfaces (Marketing, 2021).
Self-cleaning is further assisted by microbumps in the proboscis that make its surface rough (Krenn et al., 2019). Surface roughness significantly increases wettability; if the surface is hydrophobic, the roughness will increase hydrophobicity. The microbumps on the surface of the proboscis are hydrophilic and form a continuous pattern with less hydrophilic valleys. (Fig. 5) The surface wettability it causes is unusual and can be considered counterintuitive since it can be expected that the liquid would be hindered while passing through this network of valleys toward the legulae where it will enter the food canal.
However, it is surprisingly advantageous since it allows the droplets to be fixed temporarily to the hydrophilic bumps, thereby ensuring the droplets do not spread in the hydrophobic valleys. Tilting its body, the insect causes the droplets to move toward the legular bands of the proboscis. This mechanism is also beneficial for self-cleaning since it facilitates the droplets' shake off and prevents the adhesion of other non-wanted substances.
This type of surface is also called an anti-fouling surface because its properties contribute to the self-cleaning and preservation of the surface. Those surfaces are extremely important for many applications in industry and agriculture. For example, several researchers focus on super-hydrophobic surfaces for their use in fluid drag reduction, anti-biofouling surfaces in the marine environment, anti-contamination surfaces of windows for buildings and many others.
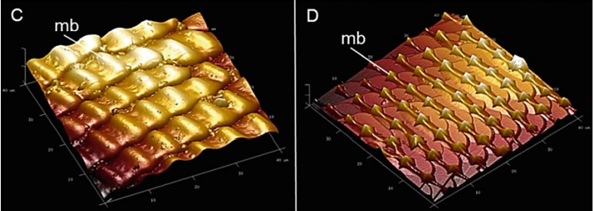
Fig. 5 Atomic force microscopy (AFM) images showing the microbumps (mb) on the galea surface of the proboscis of Papilio glaucus (c) and cabbage butterfly, Pieris rapae (d) The images represent 40 μm x 40 μm section (Krenn et al., 2019).
In addition to the wetting characteristic of the proboscis, other structural elements contribute to the self-cleaning property of the proboscis (Pometto, 2019). In particular, the arrangement of the dorsal legulae also acts as a filter. The dorsal legulae are closely spaced in the hydrophobic region of the proboscis, while they are situated more widely apart in the hydrophilic drinking portion to create slits through which the fluid enters the food canal. These slits act like filters, straining out pollen, dirt and other debris. After feeding, the lepidoptera discharges saliva through those slits, allowing any debris from the drinking region to dislodge, and thus acting as self-cleaning.
The self-cleaning properties of the Lepidoptera proboscis are closely related to the structure of the proboscis and what can be called its hydrophilic/hydrophobic dichotomy. Those characteristics are essential for the insect, which has a long proboscis and cannot use its forelimbs to actively remove debris from their mouthparts. This passive self-cleaning allows the insect to maintain critical functions of the proboscis.
Gustatory sense and response
Moths and butterflies are among many species that feed from flower nectar: a sugar-rich viscous liquid produced by the nectaries located close to the ovaries of the flower. Nectar acts as a synonymous energy source for animals and aids in plant reproduction with the transfer of male sexual material to other flowers. Thus, nectar acts as a reward for pollinators which is why it is found near the pollen, so the pollinator inevitably aids the flower in reproduction. The butterfly proboscis is the organ associated with feeding and will extend upon sensing sufficient sugar concentration in nectar (Kim, 2011). Nectar varies from concentrations of 5% to 65% sugar, compared to a coca-cola can which is 10%. Sucrose response is thus an intrinsic function of the proboscis accompanied by less efficient sugar stimulation by the legs. However, since the gustatory sensillia in the proboscis are concealed and difficult to reach when coiled up, the legs and antennae provide essential stimulation sites to incite proboscis extension (Hostachy, 2019). The receptors on the proboscis itself are used more prominently when it has been inserted in the flower and the butterfly must decide if they actually want to feed.
Nectar concentrations are unique to the flower and nectar-feeding species have concentration preferences affected by feeding techniques. A higher sugar concentration would be assumed to be optimal; however, there are drawbacks associated with high concentrations due to its viscosity (Kim, 2011). Moreover, in dryer conditions, insects may choose to prioritize diluted nectars to increase water intake. Nectar sugar concentration optimal for energy transport depends on feeding techniques employed and energy requirement (Kim, 2011). Thus, the parameters of proboscis extension response will be unique to the needs of the species, the nectar composition, and external conditions. Chemosensory sensilla are found as bristles along various parts of the butterfly, namely the proboscis, legs, and antennae. Sugar stimulation works by phagostimulant contact with these sensilla. Sensing of sugars and amino acids, which Lepidoptera tends to prefer, as well as deterrent compounds, indicates the presence of many receptor proteins on each sensory neuron (Hostachy, 2019).
Neuroanatomy of the proboscis extension response
Glossary Tastants: Experimental substance employed to stimulate a response in the taste sense. Enantiomer: A pair of molecules that are stereoisomers (same molecular composition and sequence but different orientation in space) that are non-superimposable mirror images. |
Analyzing the neuronal pathway from sensilla on the proboscis and antennae to incite the proboscis extension response aids in understanding the response to various stimuli and insect-plant coevolution. Sensilla that line many of the external organs contain terminal pores as seen in Figure 6, inside which there are gustatory and olfactory sensory neurons (Mitchel,l 1999). Each of these neurons is connected to an axon path that carries the sensory information to the central nervous system of the insect, which is then believed to be processed in the suboesophageal ganglion.
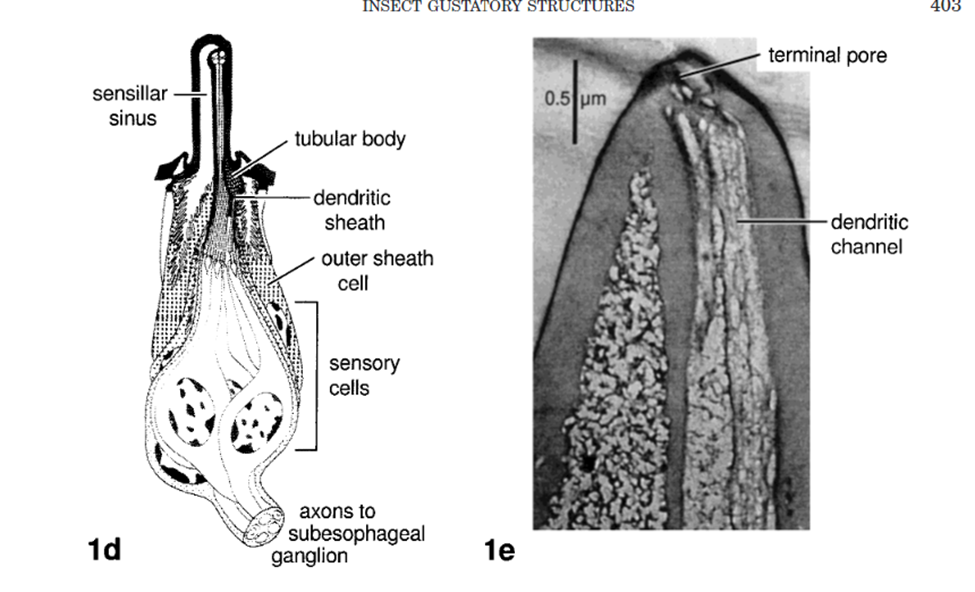
Fig. 6 Insect gustatory response structures (Mitchell 1999). 1d is a sample sensilla pore that connects external sensory neurons to transport information to the central nervous system. 1e is a photograph of a longitudinal section through a labella sensillium of P. regina with the 4 chemosensitive dendrites.
The motor neurons are then connected to the reflex of proboscis extension. The neurons located in the sensilla are both mechanosensory and chemosensory to sense contact and then determine the nectar quality (Mitchell, 1999). This duo of sensors works first by sensing the sensillum contact with the surface, activating the chemosensory pathway. Fruit flies are another species that demonstrate proboscis extension response behavior and their response to various tastants has indicated more precisely the composition of their gustatory response neurons. Four chemosensory neurons were found to each encode for various taste sensations. These senses are categorized called water cells, salt cells, and sugar cells (Popescu, 2013). Typically, the path takes gustatory information to be processed by central organs such as the brain or, in the case of M. sexta, the subesophageal ganglion (part of the nervous system responsible for regulating behavioral regulation and integrative learning). However, some species, such as N. bullata present, interneural circuitry was found to bring gustatory information directly from the contact sensilla to incite the proboscis extension response with little processing (Mitchell, 1999).
Upon analysis of the phago stimulatory neurons responding to NaCl and sucrose stimulation, the lines projected in the central nervous system confirm that the labellar response was absent for one compound and not the other and vice versa (Popescu, 2013). This confirmed the dissociation of tastant response in the fly Sarcophaga bullata and led to research in gustatory preferences and co-evolution of flowers and species with certain nutritional requirements. However, it has not been discovered how interactions of multiple favored molecules with deterrents could affect the proboscis extension response. Masking of certain compounds could occur if the orientation and sensation were favorable to it as well as synergy of favored compounds that intensifies the reflex (Popescu, 2013).
External considerations for proboscis extension response
Another approach to neural analysis is the discussion of external factors that affect proboscis extension response (PER) upon chemosensory reaction. First of all, the effects of satiety on the reflex are briefly explored. Tests were performed to determine whether there is a dissociation in the responses to gustatory sensing of nutritionally valued food as opposed to satiety satisfaction (Musso, 2017). The effects of non-caloric sweeteners on PER are explored using the non-metabolizable enantiomer of natural D-glucose: L-glucose. D glucose is the naturally found compound that has synthetically produced enantiomer L-glucose, seen in Figure 7, which is considered non-caloric as it cannot be metabolized.
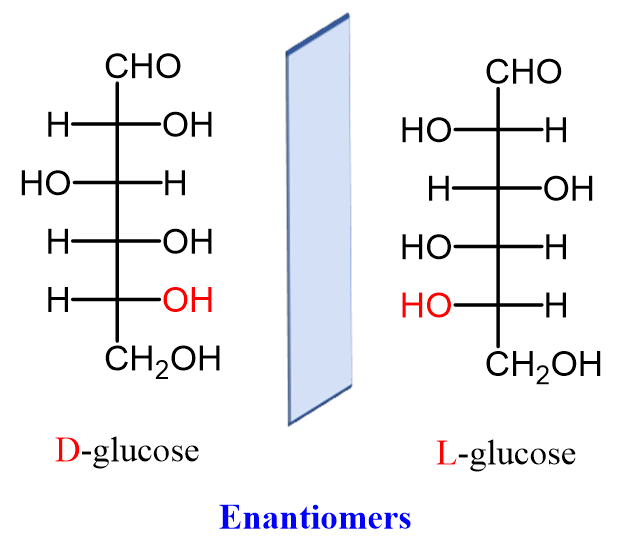
Fig. 7 Naturally occurring D-glucose and its synthetic enantiomer L-glucose (Morsch, 2020).
In typical PER experiments, subjects are treated with specific solutions used to determine short-term memory and long-term memory associations with proboscis extension response. Since the sweet taste sensed by gustatory receptors is linked to energy gain, the insect will have the PER reflex. However, in the absence of this energy gain in non-caloric sweeteners, the species will form Caloric Frustration Memory (CFM) and show reduced sugar response as a result (Musso, 2017). Thus, satiety modulated by dopamine release is prioritized as the insect still instinctively has a PER demonstrating an external motivator to extend the proboscis. Whether hunger is still a factor in gustatory response to elicit a PER will be further explored. Nonetheless, this brings up another consideration which is the effects of dopamine in modulating PER.
The feeding regulation of the species Drosophila, the fruit fly, has been tested for which modulatory neurons are responsible for proboscis extension response. Genetically identical flies are treated with an inward-rectifying potassium channel which prevents membrane depolarization. It was thereafter noticed that TH-Gal4 neuron lines showed a decrease in extension probability with a lower sucrose sensitivity (Marella, 2012). The neurons that express the rate-limiting enzyme in the dopamine production pathway are marked by TH-Gal4, thus indicating dopaminergic modulation of the proboscis extension response. This enzyme, known as tyrosine hydroxylase, plays a vital role in feeding, with exposure resulting in a failure to feed. Figure 8 C shows the striking effects of treatment with Kir2.1 that express tyrosine hydroxylase and limit PER. This notion indicates that even with proper chemosensory of sugar concentrations and hunger, dopamine pathways are required to initiate the motor response.
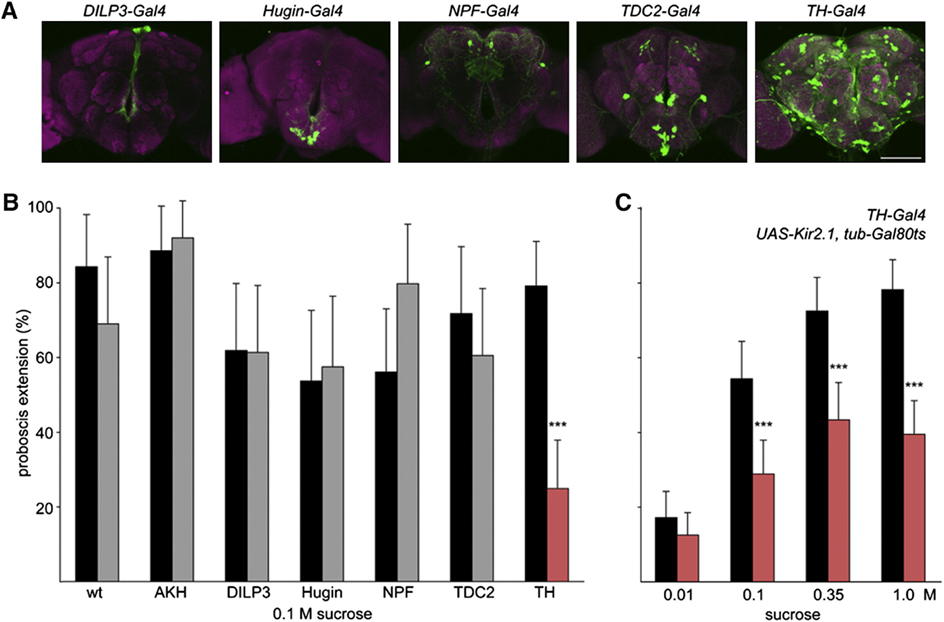
Fig. 8 (A) Each neuron is labeled with GFP and corresponding activity is observed for a PER. (B) Neural activity difference for Kir2.1 treated potassium channels (gray) versus non-treated (black) during a PER with 0.1M sucrose solutions. (C) Treated flies show reduced proboscis extension response for a range of sucrose concentrations (Marella, 2012).
Further testing showed a positive correlation between changes in dopaminergic activity and extension response. However, it is discussed that all of these tests were performed on Drosophila in a starving state. Thus, when further examining the neuroanatomy of the gustatory response pathways, TH-VUM, a single dopaminergic neuron, is investigated (Marella, 2012). This neuron is not directly involved in taste response but a longer-term responder to cues such as satiety state, which reinforces that animals feed based on the requirement. The animal's nutritional needs will carry information through TH-VUM to promote extension response and will deter feeding when satiety is achieved (Marella, 2012).
Bees unusual attachment to sugar
Bees, similar to butterfly moth and fly species, have chemosensory receptors that activate a PER to feed and pollinate. As similar as they are in gustatory response, bees have been observed to have significantlylonger and more intense neural activity to sugar sense (Miriyala, 2018). The bumblebee is the species that research has delved into the neuroanatomical reasoning behind longer-than-usual feeding from nectar sources. The first sensation of sucrose causes a spike in chemical and neural response in all insects but the presence of a second sugar-activated neuron contributes to the abnormally intense response (Miriyala, 2018). The neurons have different response behavior, one responsible for the initial activity and the second burst firing gustatory receptor spikes as seen in the graph in Figure 9 which allows for sustained activity. This is explored as an evolutionary step for honeybees to not only forage nectar for themselves but also to bring it back to the colony and store it for the hive. Similar adaptations occur all over the range of species relying on gustatory sensilla for feeding response. This introduces the notions of preference and adaptive evolution for species that favor certain feeding substrates.
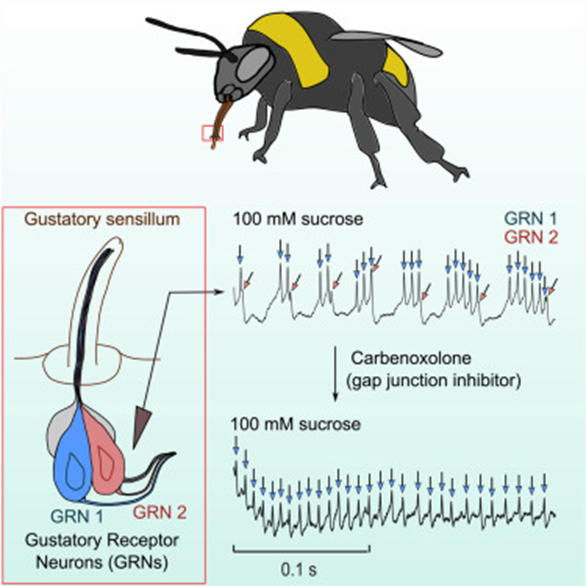
Fig. 9 The neuroanatomy of the honeybee accompanied by graphical interpretations of burst firing Gustatory Receptor Neurons (GRNs) (Miriyala, 2018).
Taste preferences in proboscis possessing species
Sugar is an important feeding stimulus in Lepidoptera and is essential to the survival of these insects, helping them maintain healthy energy supplies. However, moths and butterflies tend to accept particular types of sugars over others. Behavioral tests reveal a clear preference hierarchy for sugars in the butterfly species.
In these tests, the taste preference is observed in 25 male and 25 female butterflies. First, the proboscis is carefully extended and dipped into one sugar solution droplet (1 μl). Then, the proboscis is held for a maximum of 10 seconds to ascertain whether absorption occurs. This process is performed with another droplet of a different sugar solution until the butterfly accepts one or rejects both. The solutions tested contain sucrose, glucose, fructose, and maltose; each with the same concentration (10% vol.) in demineralized water (Dierks & Fischer, 2008).
Results reveal an increase in positive response in correlation with increased sugar concentration. Specifically, butterflies prefer sucrose over glucose, fructose, and lastly maltose, in decreasing order (Fig. 10) (Dierks & Fischer, 2008).
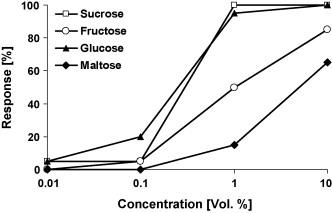
Fig. 10 Feeding response of Bicyclus anynana to four different sugars in increasing concentration (Dierks & Fischer, 2008).
While sugar is an important feeding stimulus in Lepidoptera, certain nutrients, such as alcohol, also precipitate a feeding response when they come into contact with the proboscis.
The same procedure for sugars is conducted with alcohols with three treatment groups: hungry, hungry but antenna-ectomized, and sugar-satiated butterflies. The solutions tested contain methanol, ethanol, propanol, and butanol; each with the same concentration (10% vol.) in demineralized water (Dierks & Fischer, 2008).
Similarly, results reveal an increase in positive response in correlation with an increase in alcohol concentration. Moreover, hungry butterflies demonstrated a stronger response in comparison to hungry but antenna-ectomized and sugar-satiated butterflies. Notably, methanol tends to be the least preferred alcohol in all three treatment groups (Fig. 11) (Dierks & Fischer, 2008).
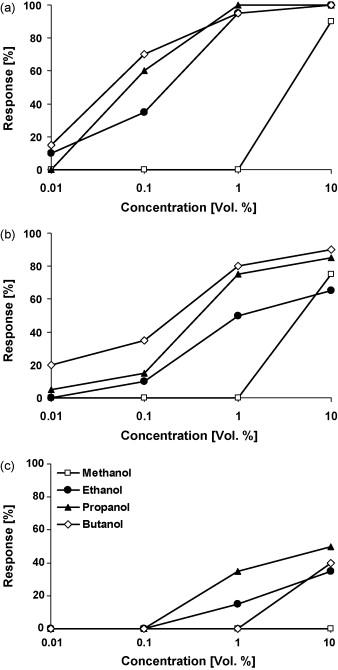
Fig. 11 Feeding response of (a) hungry, (b) hungry but antenna-ectomized, (c) sugar-satiated Bicyclus anynana to four different alcohols in increasing concentrations (Dierks & Fischer, 2008).
In general, the Lepidoptera prefers nectar rich in amino acids rather than plain nectar lacking in proteins. Amino acids play an important role during mating, as males require a protein-rich diet in order to transfer nutrients to females during copulation. These nutrients contribute to vitellogenesis, help with the female's somatic maintenance and, most importantly, are used during egg production (Erhardt, 1992).
Outside of insects, other proboscis-possessing species have similar taste preferences for amino acids, with sugars as a preferred macromolecule. In particular, studies on musk shrews (Fig. 12), which are insectivores and feed on a variety of insects and worms, reveal a preference for protein-rich materials.
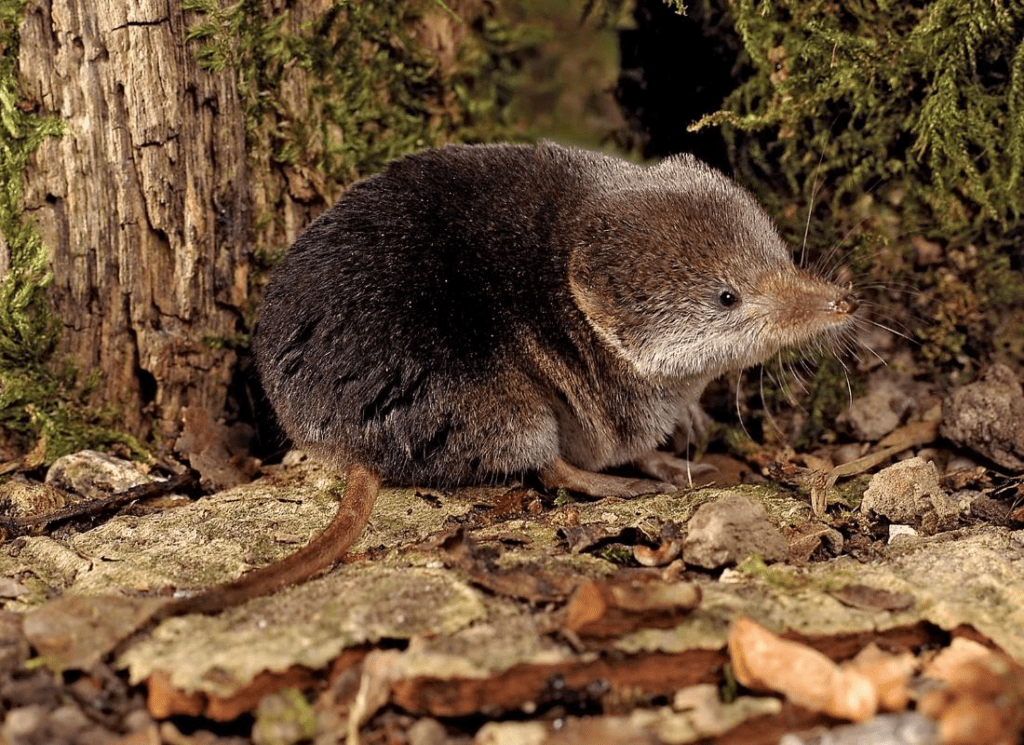
Fig. 12 Common Eurasian musk shrew (Sands, 2010).
In these studies, preference testing is observed in 20 adult house musk shrews of equal sexes. These small mammals are housed in a box containing two polyethylene drinking tubes of 100 ml, one consisting of deionized water and the other consisting of an amino acid solution. The test is conducted over a period of 48 hr, with the position of the drinking tubes changed after 24 hr. The solutions tested contain NaCl, sucrose, quinine, HCl, and saccharin Na (Iwasaki & Sato, 1982).
Results reveal a significant preference towards sucrose, while other amino acids are rejected at varying amounts. Thus, shrews demonstrate high sensitivity toward bitter-tasting compounds (Fig. 13) (Iwasaki & Sato, 1982).
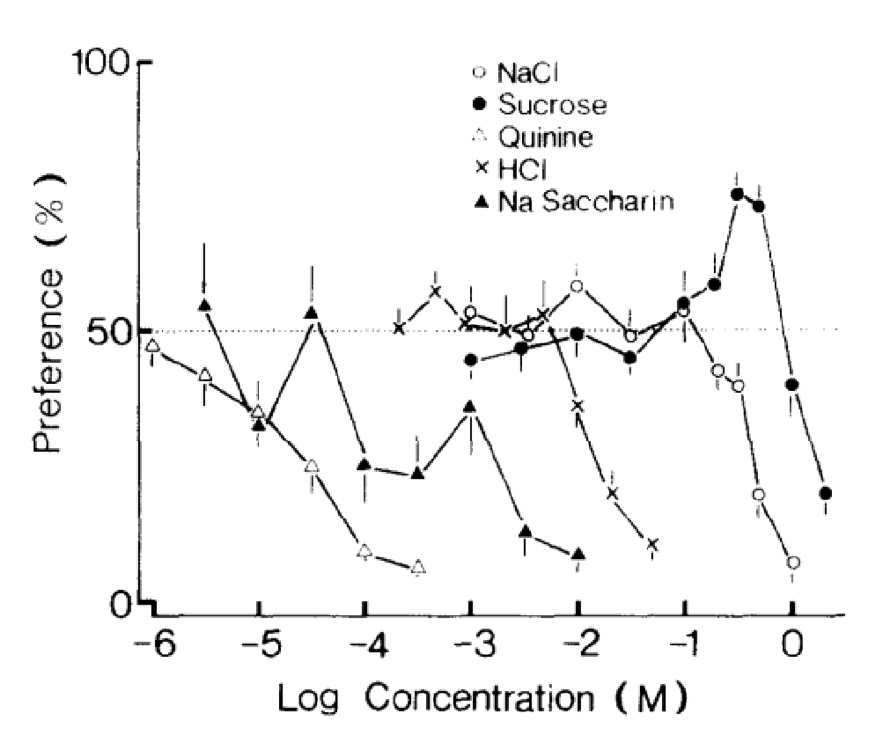
Fig. 13 Taste preference for different concentrations of NaCl, sucrose, quinine, HCl and saccharin Na in house musk shrews (Iwasaki & Sato, 1982).
Another notable example is the elephant, a herbivore that feeds on a wide range of plants and fruits. Similarly, behavioral tests reveal a taste preference towards sugar-rich fruits.
African elephants have an incredibly keen sense of smell, as their olfactory system is composed of around 2000 different functional olfactory genes. These mammals can recognize fruit sugar content based on olfactory cues alone. In an odor-based choice behavioral experiment, African elephants are presented with two different types of fruits without being able to visibly see them.
Results reveal a stronger preference for sugary fruit. As the proportional difference in sugar content (ratio of % sugar between a pair of fruits) decreases, i.e. the rank difference in sugar content between the two fruits increases, the proportion of elephants selecting the higher sugar option increases as well (Fig. 14) (Nevo et al., 2020).
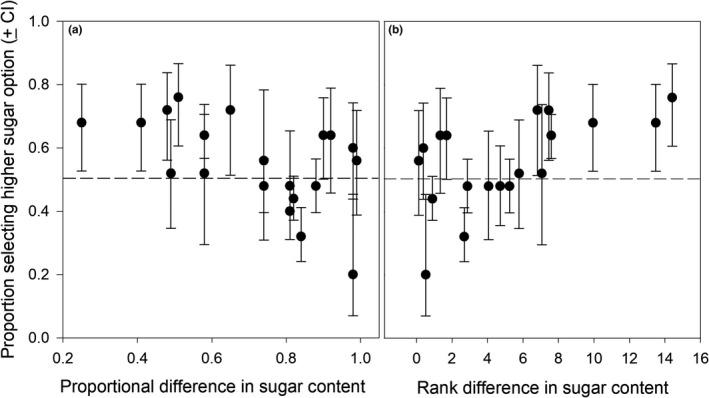
Fig. 14 Proportion of elephants selecting the fruit with high sugar content as a function of (a) proportional difference in percent sugar content, and (b) rank difference in percent sugar content between pairs of fruits (Nevo et al., 2020).
Therefore, among proboscis-possessing species, insects such as butterflies and moths exhibit a similar taste preference when compared to mammals such as musk shrews and elephants. These species have a strong preference for sucrose, suggesting a connection between the structure and function of the proboscis in relation to the role of sugar in their survival.
Mosquito proboscis
Unlike the majestic butterfly, mosquitoes are often disliked because of their tendency to carry and spread diseases through irritating bites. However, what the butterfly and mosquito do have in common is their proboscis, a homologous organ due to the similar basic structure; they only differ slightly due to their different functions (Phylum Arthropoda …, 2018), which will be elaborated further in the paper.
The microneedle-like structure
The proboscis of a mosquito is one of the three types of appendages found on the head of most dipterans, the order of insects containing many types of flies and mosquitoes; the other two types of appendages, the antenna and the maxillary palps (palps attached to the jaw or jaw bone), work alongside the proboscis to complete the critical function of feeding. Figure 15 below is an image that depicts these parts on an adult mosquito.
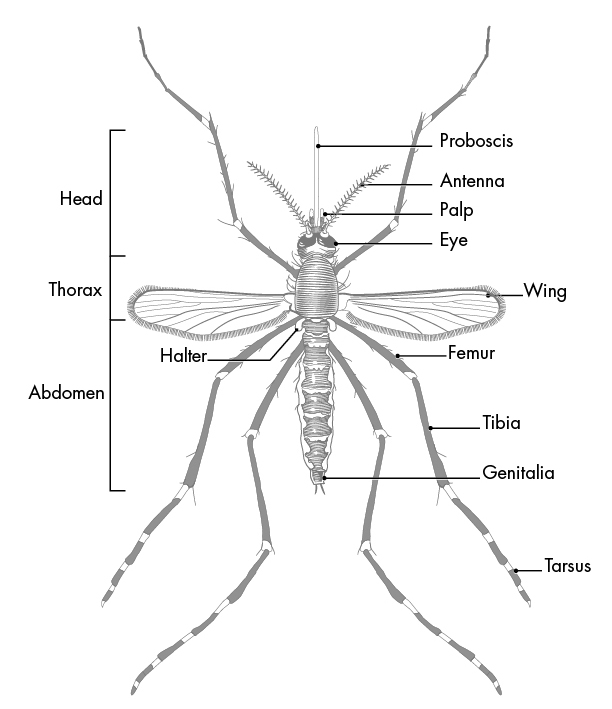
Fig. 15 Parts of an Adult Mosquito (What is a mosquito?, 2020).
Although both male and female mosquitoes have proboscis, their appearance and structure slightly differ due to differing primary functions; the male mosquito's primary focus is mating, whereas the female mosquito's primary focus is reproducing. As such, the male's proboscis is much wider, branched and feathery, allowing it to detect the buzz of a female during mating, while the female's proboscis is much less feathery and more needle-like, allowing it to pierce an animal's skin and obtain the necessary nutrients for reproduction found in blood (Male vs. Female Mosquitoes: What's the difference?, 2020). Indeed, it is the female mosquito that we are concerned with as humans since they are the ones that cause the annoying itchy bite, so female mosquitoes will hereafter be the focus of this section.
In order to pierce skin, the female proboscis has a much more sophisticated structure. Although mosquitoes seem to be piercing the skin with one needle-like structure to the human eye and touch, research has shown that the proboscis is actually a microneedle-like structure composed of 6 needles, known as stylets, each piercing the skin to help acquire blood in a different way. Stylet is a term used to describe a mouthpart that actually pierces the skin, and a group of stylets is known as a fascicle (How Mosquitoes Bite, n.d.). The six stylets include a pair of sharp saw-like needles known as maxillae (Fig. 16A), a pair of needles known as mandibles, a gutter-like needle known as the labrum (Fig. 16B), which is covered by the last stylet, the hypopharynx, to form a tube-like structure. The fascicle is then enclosed by the labium, which has two lobes on the tip known as labella (Choo et al., 2015). Figure 17 summarizes the structure of a female mosquito proboscis, from the six stylets to the labium and labella.
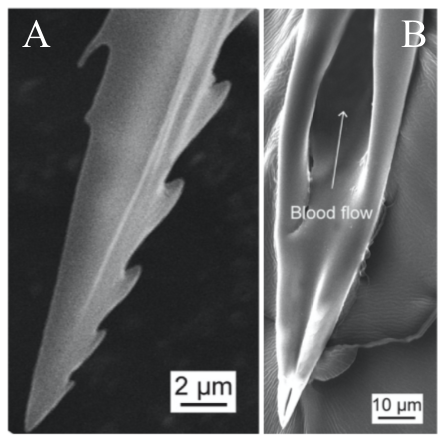
Fig. 16 Scanning electron micrographs (SEM) of (A) the saw-like teeth of maxillae and (B) the sharp needle and tube structure of the labrum (Gurera et al., 2018).
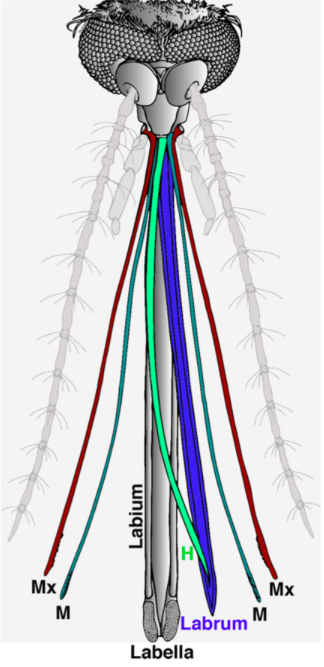
Fig. 17 Illustration of the 6 microneedle-like structure of a female mosquito proboscis used to pierce skin for blood. The Mx cayenne colored pair of needles is the maxillae, the M fern colored pair is the mandibles, the H clover colored needle is the hypopharynx, and the labrum is colored in blue; the labium and labella are both shown in gray (Choo et al., 2015).
Mosquito feeding mechanism
Although mosquitoes are generally only viewed as blood-sucking insects, they are pollinators as well! The fundamental nutrients needed for adult mosquitoes are found in the nectar of flowers, so mosquitoes spend most of their time pollinating flowers (Fig. 18) rather than feeding on blood; this is the most overlooked ecological function of mosquitoes since they tend to only pollinate flowers near or after dusk away from human disturbance (The bizarre and ecologically important hidden lives of mosquitoes, 2019). While both female and male mosquitoes need nectar to survive, female mosquitoes will also feed on the blood of vertebrate hosts as it contains the nutrients necessary to lay fertilized eggs.
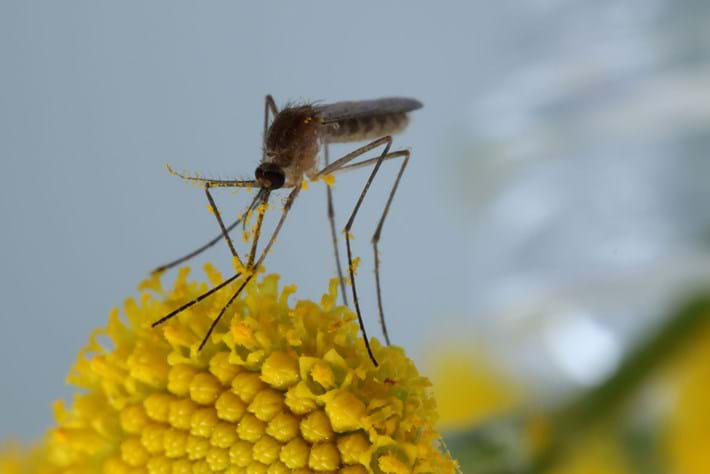
Fig. 18 A pollinating Culex pipiens covered in tansy pollen (The bizarre and ecologically important hidden lives of mosquitoes, 2019).
It has recently been suggested that the proboscis may act as a sensory organ, like the antennae, as it is involved in the reception of chemical cues and environmental signals. As such, after the mosquito lands on a host, it will use the olfactory and gustatory sensilla on the labella to locate an area containing blood vessels, and once the fascicle has pierced the skin, the labrum continues to probe around and change its orientation to precisely find the blood vessels using one of the protein receptors found on its proboscis that react to volatile chemicals in blood (Quiros, 2016). If a mosquito were to randomly pierce an area of skin, it would have a very low probability of finding blood, demonstrating the importance of the sensors in the proboscis (Choo et al., 2015). Figure 19 below shows an image of a mosquito proboscis probing for blood vessels.
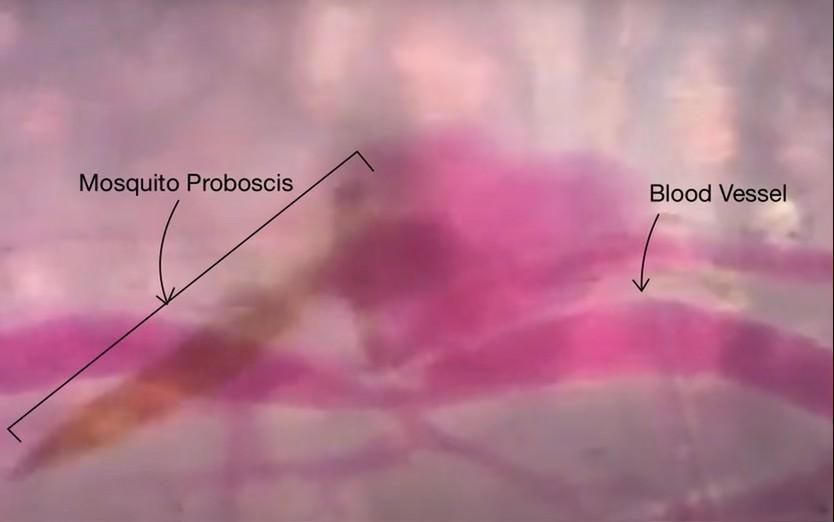
Fig. 19 Image of a mosquito proboscis probing the skin for blood vessels (Deep Look, 2016).
For female mosquitoes to obtain the nutrients in vertebrate blood, the different parts of its proboscis work together effortlessly and painlessly to pierce skin using a force of three orders of magnitude less than artificial microneedles. First, the fascicle is inserted into the skin with the help of the two mandibles holding the skin apart on each side, as well as the cooperation between the sharp labrum and the sawing motion of the serrated maxillae at a vibration frequency of 15 Hz; the labium does not enter the skin; instead it slides over the fascicle with the labella maintaining its contact with the outer layer of the skin. As the fascicle enters the skin, the hypopharynx releases its saliva containing numbing agents under the skin that act as anesthetics to block the signaling between pain receptors and the brain. Mosquitoes' salivary glands contain more than 1000 different compositions of proteins, each with different functions; two families of proteins, anosphensin and anophelin will be covered in this paper. The anosphensin family of proteins is responsible for numbing; they inhibit the growth of a pain-inducing peptide known as bradykinin. The numbing agents combined with the reduced insertion force caused by the vibratory motion of the maxillae allow the mosquito to cause less tissue deformation, which reduces nerve sensation and makes the bite painless (Gurera et al., 2018). Figure 20 below summarizes the main points of the first step of a mosquito's feeding process.
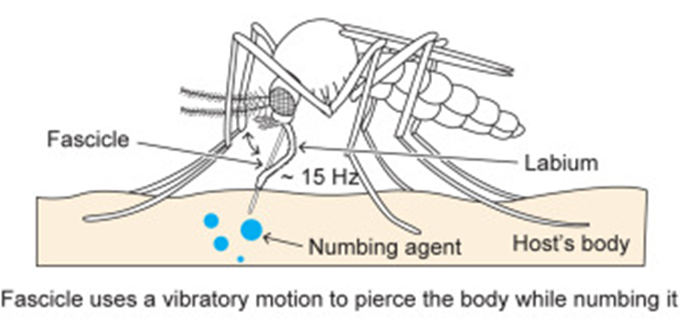
Fig. 20 The first step of the mosquito feeding process: the piercing of the skin, the sliding back of the labium, and the release of numbing agents (Gurera et al., 2018).
Once the skin has been pierced and a blood vessel is detected, the sharp tip of the labrum pierces the blood vessel with a lowered vibration frequency of 5 Hz, since the material is much softer, and blood is withdrawn through the labrum and hypopharynx tube (Fig. 21). As the mosquito's abdomen fills with blood, the water in the blood is separated from the red blood cells and excreted through its rear end; this allows the mosquito to hold a much larger content of red blood cells, where all the protein is found.
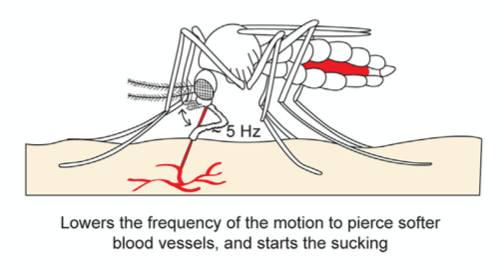
Fig. 21 The second step of the mosquito feeding process: the piercing of the blood vessel, the blood suction, and the separation of water from the red blood cells (Gurera et al., 2018).
Finally, once the abdomen is completely filled with blood, the mosquito retracts the fascicle from the skin and back into the labium while, once again, releasing saliva from its hypopharynx (Fig. 22); this time, the proteins of the anophelin family are secreted to prevent blood clotting by binding to the blood coagulation enzyme thrombin. Because blood tends to coagulate upon contact with air, the mosquito releases these chemicals to keep the blood flowing. The use of its saliva is therefore important in remaining unnoticeable from the numbing in the beginning to the prevention of blood coagulation at the end. As such, researchers have taken an interest in studying the mosquito's saliva, as it could be the key to developing new drugs to treat deep vein thrombosis or stroke (Mosquito saliva vital to the discovery of future drugs, 2018).
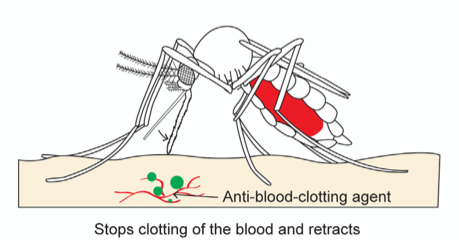
Fig. 22 The third and final step of the mosquito feeding process: the retraction of the fascicle back into the labium and the release of anti (Gurera et al., 2018).
Host detection
Through the three-step feeding process, mosquitoes on the skin detect blood vessels through its many sensory organs on the labrum and the labella. However, how do mosquitoes choose which host to land on in the first place? It turns out, mosquitoes have more than 150 protein receptors on their head appendages, which compose the primary olfactory system, to detect the presence of nearby hosts. These receptors primarily detect the presence of carbon dioxide, which is exhaled by all living organisms, heat, moisture, certain chemicals that produce body odor, such as lactic acid and ammonia, and volatile fatty acids on the surface of the skin; this makes individuals that do certain activities that increase breathing more susceptible to attracting mosquitoes as it increases the amount of carbon dioxide exhaled and the production of sweat (Mosquitoes: Born from blood, 2022). The mosquito first detects the carbon dioxide exhaled, at a distance of more than 30 feet (Greenfieldboyce, 2019); then, as it gets closer to the host, it detects body odors and volatile acids, and finally heat. The receptors to carbon dioxide are found on the nerve cells called cPa neurons (How Mosquitoes Detect People, 2013). In a study where each head appendage of Anopheles stephensi was removed and host detection was measured, the proboscis was found to be the main head appendage involved in the sensory activity of host detection (Fig. 23).
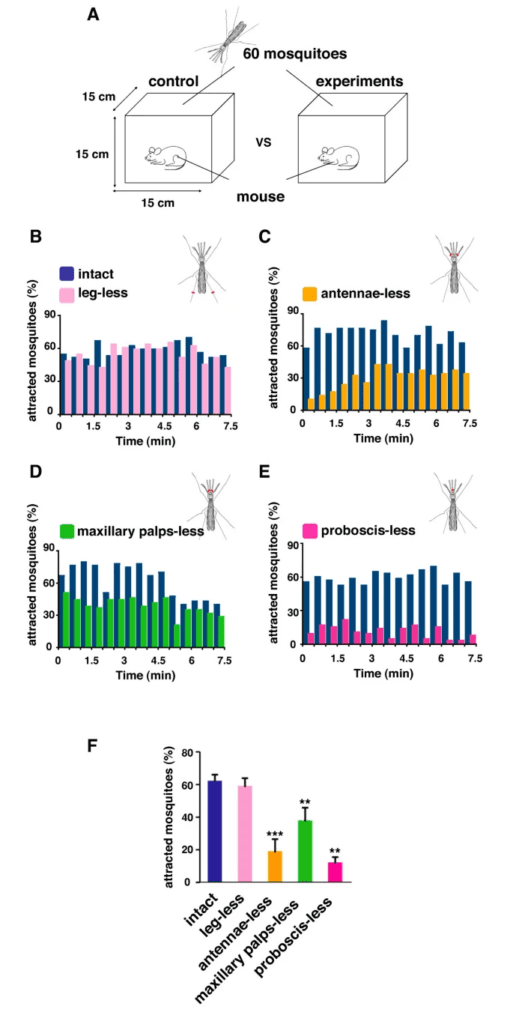
Fig. 23 Summary of mosquito host detection study. (A) Schematic of the host detection assay. The study used 120 female mosquitoes and mice as the host. The control was a group of mosquitoes with none of its appendages removed. Host detection was measured by counting the number of blood sucking mosquitoes on (B) leg-less mosquitoes, (C) antennae-less mosquitoes, (D) maxillary palps-less mosquitoes, and (E) proboscis-less mosquitoes. (F) Comparison of mosquito activity as each appendage was removed, with the proboscis-less mosquitoes displaying the lowest level of activity because host detection was impaired (Maekawa et al., 2011).
Conclusion
In summary, the proboscis is essential for fluid feeding insects like bees, butterflies or mosquitoes. Its physical and chemical properties allow the insect to perform critical functions like foraging, mating and feeding. To maintain these abilities, the proboscis has self-cleaning properties related to its structure and wettability. The hydrophobic and hydrophilic regions of the proboscis play a role in preventing debris or bacteria contamination, thus preserving the proboscis. One of the key functions of proboscis is assisted by chemosensory proteins, which play a vital role in proboscis sporting animals before they perform the almost magical mechanisms of unfurling. Sensory receptors all over the body work synergistically to provide neural responses to determine whether feeding is desired and where the best source of food would be found. Factors that affect proboscis extension response include the gustatory preference of the species, the quality of nectar detected, modulating chemicals in the species and many still unknown neuroanatomical conditions. In addition, proboscis-possessing species tend to prefer sucrose over other sugars including fructose, glucose, and maltose. Overall, sucrose is the preferred source among other solutions, including alcohols and various amino acids.
References
The bizarre and ecologically important hidden lives of mosquitoes. (2019, December 5). Earth Touch News Network. https://www.earthtouchnews.com/natural-world/how-it-works/the-bizarre-and-ecologically-important-hidden-lives-of-mosquitoes/
Chen, D., Song, H., Liu, Q., Gan, J., Liu, Y., Chen, K., Wang, C., Wen, S., Zhou, Y., Yan, C., Zhang, J., Shi, Y., Han, Z. (2020). Large Curvature Folding Strategies of Butterfly Proboscis. Journal of Bionic Engineering, 17, 1239-1250. https://doi.org/10.1007/s42235-020-0089-1
Choo, Y., Buss, G. K., Tan, K., Leal, W. S. (2015). Multitasking roles of mosquito labrum in oviposition and blood feeding. Frontiers in physiology, 6(OCT), 306. https://doi.org/10.3389/fphys.2015.00306
Deep Look. (2016, June 7). How Mosquitoes Use Six Needles to Suck Your Blood | Deep Look. [Video]. Youtube. https://www.youtube.com/watch?v=rD8SmacBUcU
Dierks, A., & Fischer, K. (2008). Feeding responses and food preferences in the tropical, fruit-feeding butterfly, Bicyclus anynana. Journal of insect physiology, 54(9), 1363–1370. https://doi.org/10.1016/j.jinsphys.2008.07.008
Erhardt, A. (1992). Preferences and Non-Preferences for Nectar Constituents in Ornithoptera priamus poseidon (Lepidoptera, Papilionidae). Oecologia, 90(4), 581–585. http://www.jstor.org/stable/4220016
Greenfieldboyce, N. (2019, March 28). How Mosquitoes Sniff Out Sweat to Find Us. npr. https://www.npr.org/sections/health-shots/2019/03/28/706838786/how-mosquitoes-sniff-out-human-sweat-to-find-us
Gurera, D., Bhushan, B., Kumar, N. (2018). Lessons from mosquitoes' painless piercing. Journal of the Mechanical Behavior of Biomedical Materials, 84, 178-187. https://doi.org/10.1016/j.jmbbm.2018.05.025
Hostachy, C., Couzi, P., Hanafi-Portier, M., Portemer, G., Halleguen, A., Murmu, M., Deisig, N., et. al. (2019). Responsiveness to Sugar Solutions in Moth Agrotis Ipilson: Parameters Affecting Proboscis Extension. Frontiers in Physiology 10:1423. https://doi.org/10.3389/fphys.2019.01423
How Mosquitoes Bite. (n.d.). Mosquito Magnet. https://www.mosquitomagnet.com/articles/how-mosquitoes-bite
How Mosquitoes Detect People. (2013, December 16). NIH. https://www.nih.gov/news-events/nih-research-matters/how-mosquitoes-detect-people
Iwasaki, K., & Sato, M. A. (1982). Taste preferences for amino acids in the house musk shrew, Suncus murinus. Physiology & behavior, 28(5), 829–833. https://doi.org/10.1016/0031-9384(82)90199-8
Kim, W., Gilet, T., Bush, J.W.M. (2011). Optimal concentrations in nectar feeding. Journal…. 108(40), 16618-16621.https://doi.org/10.1073/pnas.110864210
Krenn, H. W. (2019). Insect mouthparts: Form, function, development and performance. Springer Nature.
Lehnert M. S., Monaenkova D., Andrukh T., Beard C. E., Adler P. H., Kornev K. G.
(2013). Hydrophobic–hydrophilic dichotomy of the butterfly proboscis. J. R. Soc. Interface.102013033620130336 http://doi.org/10.1098/rsif.2013.0336
Maekawa, E., Aonuma, H., Nelson, B., Yoshimura, A., Tokunaga, F., Fukumoto, S., Kanuka, H. (2011). The role of proboscis of the malaria vector mosquito Anopheles stephensi in host-seeking behavior. Parasites & Vectors. 4(10). https://doi.org/10.1186/1756-3305-4-10
Male vs. Female Mosquitoes: What's the difference? (2020, November 18). MosquitoNix. https://mosquitonix.com/blogs/news/male-vs-female-mosquitoes-whats-the-difference
Marella, S. Mann, K., & Scott, K. (2012). Dopaminergic Modulation of Scurose Acceptance Behaviour in Drosophila. Neuron, 73(5), 941-950. https://doi.org/10.1016/j.neuron.2011.12.032.
Marketing, I. (2021, February 24). The science of hydrophobicity. IGL Coatings Blog. https://blog.iglcoatings.com/the-science-of-hydrophobicity/
Miriyala, A., Kessler, S., Rind, F.C., & Wright, G.A. (2018). Burst Firing in Bee Gustatory Neurons Prevents Adaptation. Current Biology, 28(10), 1585-1594, https://doi.org/10.1016/j.cub.2018.03.070.
Mitchell, B.K., Itagaki, H., & Rivet, M-P. (1999). Peripheral and central structures involved in insect gustation. Microscopy Research and Technique, 47(6), 401-415. https://doi.org/10.1002/(SICI)1097-0029(19991215)47:6<401::AID-JEMT4>3.0.CO;2-7
Morsch, L. (2020). R and S Assignment in Compounds with Two or More Stereogenic Centers. LibreTexts Chemistry. https://chem.libretexts.org/Courses/University_of_Illinois_Springfield/UIS%3A_CHE_267_-_Organic_Chemistry_I_(Morsch)/Chapters/Chapter_05%3A_Stereochemistry/4.07%3A_R_and_S_Assignments_in_Compounds_with_Two_or_More_Stereogenic_Centers
Mosquito saliva vital to the discovery of future drugs. (2018, March 29). The University of Sydney. https://www.sydney.edu.au/news-opinion/news/2018/03/29/mosquito-saliva-vital-to-the-discovery-of-future-drugs.html
Mosquitoes: Born from blood. (2022, September 19). Science Boreal. https://blog.scienceborealis.ca/mosquitoes-born-from-blood/
Musso, P-Y., Lampin-Saint-Amaux, A., Tchenio, P., & Preat, T. (2017). Ingestion of artificial sweeteners leads to caloric frustration memory in Drosophila. Nature Communications, 8, 1803. https://doi.org/10.1038/s41467-017-01989-0
Nevo, O., Schmitt, M. H., Ayasse, M., & Valenta, K. (2020). Sweet tooth: Elephants detect fruit sugar levels based on scent alone. Ecology and evolution, 10(20), 11399–11407. https://doi.org/10.1002/ece3.6777
Pometto, Suellen Floyd. (2019). Self-Repair and Self-Cleaning of the Lepidopteran Proboscis. All Dissertations. 2452. https://tigerprints.clemson.edu/all_dissertations/2452
Phylum Arthropoda: Insect mouthparts (Butterfly, cockroach, housefly, honey bee, Mosquito). (2018, September 3). StudyandScore. https://www.studyandscore.com/studymaterial-detail/phylum-arthropoda-insect-mouthparts-butterfly-cockroach-housefly-honey-bee-mosquito
Popescu, A., Couton, L., Almaas, TJ. et al. (2013). Function and central projections of gustatory receptor neurons on the antenna of the noctuid moth Spodoptera littoralis . Journal of Comparative Physiology, 199, 403–416. https://doi.org/10.1007/s00359-013-0803-0
Quiros, G. (2016, June 7). How Mosquitoes Use Six Needles to Suck Your Blood. KQED. https://www.kqed.org/science/728086/how-mosquitoes-use-six-needles-to-suck-your-blood
Sands, A. (2010). Common Eurasian shrew (Sorex araneus) [Photograph]. Nature Picture Library.
What is a mosquito? (2020, March 5). Centers for Disease Control and Prevention. https://www.cdc.gov/mosquitoes/about/what-is-a-mosquito.html