Cephalopods and their Beak
Palmyra Mendoza Cabrer, Karina Carlson, Rylee Mcdonald, Michael Weldon
Introduction
From ants to elephants — and passing through sea cucumbers, adaptations in the animal kingdom are majestically diverse. For instance, means of travel include legs, more legs, wings, flippers and suction cups. Bodies can be scaled, slimy, furry, feathered, spiky, or armored and the list goes on. Basic feeding can involve teeth, pincers, protruding tubes, external stomachs (Fig. 1) or classic beaks.
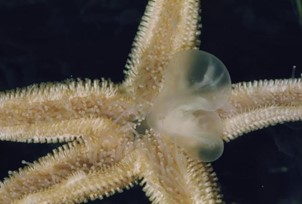
Hence, there is incredible variation, even between animals that share characteristic features. Take birds for instance, these feathered flyers share many traits but analyzing a single one — the beak for instance, reveals a panoply of species-specific specializations. But it does not stop there; beaks have many more uses than those that first come to mind. From the golden eagle — king of the skies, to giant squids — titans of the deep, beaks are integral to the lives of many creatures. While the former is no doubt fascinating source material for a research project, the latter is less well-known and arguably even more impressive. This report presents the brilliant principles behind the cephalopod's beak designs.
Cephalopods are a class of mollusk (Roper and Voss, 2020), so they are related to snails and clams, but today's subjects are much more interesting (in the traditional, first-impression sense at least). Cephalopods include octopuses (Fig. 2), nautiluses (Fig. 3), squids (Fig. 4), and cuttlefish (Fig. 5) (Roper and Voss, 2020).
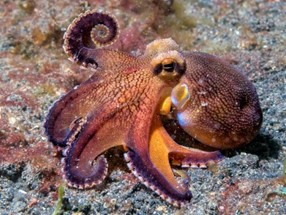

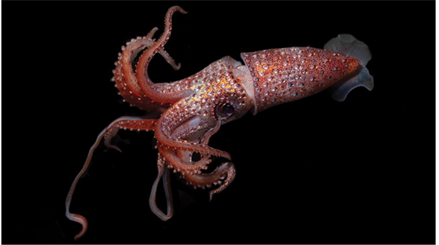
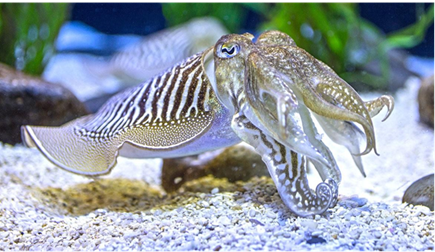
The report aims to analyze their beak, but with a particular focus on its integration into the animals' bodies and lifestyles. Thus, it will begin with a brief description of cephalopods before presenting their beak and will then return to the organisms — to explore how well suited the feature is to them. The initial presentation of cephalopods will establish the range their basic beak design may accomplish, through stating the range of shapes and sizes of the animals as well as their prey and hunting strategies. With that understood, the beak itself will be presented. In particular, its structure and composition — truly a marvelous affair. As cephalopod babies are so different from what they will become, the growth process of the beak throughout aging will also be touched upon. This should all communicate the idea that animals are biological systems, and the cephalopod beak is just one component, a component beautifully integrated with its host. To wrap things up, some potential applications for the beak's technology will be presented.
Cephalopods
As seen in Fig. 2 to 5, these beautiful creatures share a general body shape. It seems there is a head, and from it sprout tentacles. These look like legs for the octopus, a skirt for the squid, a beard for the cuttlefish and not quite any of those for the nautilus. This general similarity is where their name comes from; cephalopod means head-foot in Greek (kephalē means head and pous (suffix –pod) means foot) (Harper, n.d.).
Body Composition
Within this “head” lie the organs, covered by an outer layer of skin called the mantle (Darling, 2016). Unusually, for non-insect-sized creatures, bone structure is lacking — cephalopods have no spine (“mollusk” | Cambridge Dictionary, n.d.). Some cephalopods have external shells that protect the mantle, like the nautilus. Some have internal shells that lie within the mantle, like cuttlefish and squids, and some have no shells at all, like octopuses. The internal shell of squid is called a pen. It is flexible and made of chitin, and it serves to support muscular tissues and organs (Weaver, 2012). Despite their shells, be they outer, inner or nonexistent, all cephalopods have a very soft body composed of muscle, organs and soft tissues. Having such soft bodies is what allows some cephalopods to squeeze (refer to Cardman, 2011 in Appendix) through ridiculously tight spaces (Hall, 2018). In fact, the only limiting factor for octopuses is the beak itself — any hole larger than their beak and the animal can get through (“Ever heard that an octopus can squeeze through any hole that its beak can fit through?” | themindcircle, n.d.).
Size
Cephalopods thrive across a variety of niches, which vary in part due to size, as cephalopod size is not a constant. The smallest squids are the Idiosepius squids, where females measure 2.5 cm in length and males, 1.6 cm (Runck, 2018), from the top of the head to the tip of the tentacle. That is about the length of a dandelion petal. The largest – the giant squid Architeuthis – can span over 20 meters (Roper and Voss, 2020). That is 3 to 4 giraffes tall. Octopuses can also reach impressive sizes. The tiniest one, octopus wolfi (Fig. 6), measures about 2.5 cm when full grown while octopus dofleini (Fig. 7) can have arm spans of 5 meters (“7 Small Octopus Species That You'll Love” | Octolab.tv, 2019). One specimen's arm span was even recorded at 9 meters (Roper and Voss, 2020). With so many species, the sizes of these animals vary greatly between those bounds. Nautiluses and cuttlefish are more consistent. For the former (Fig. 8), adult shells measure between 16 and 21 cm in diameter (“Chambered nautilus” | Monterey Bay Aquarium, n.d.). Cuttlefish length varies within 15 and 25 cm, though one species regularly reaches 50 cm in mantle length (body, excluding tentacles) (Reid et al., 2005). Thus, the diets of these creatures cannot be the same, given the huge difference in necessary caloric intake. To add to that conclusion, several species eat other cephalopod species (Hanlon and Messenger, 1996), and for some squids, that includes their own (Yong, 2008).
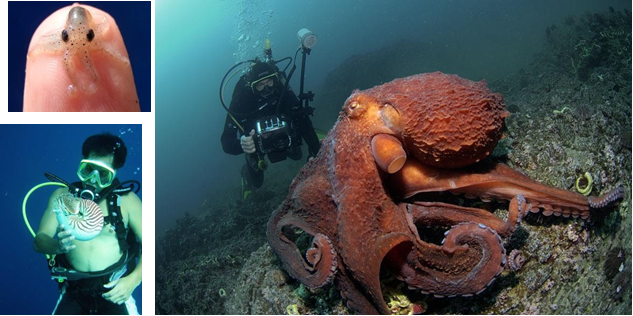
Fig. 7 Pacific Giant Octopus. [Adapted from “Octopus Valentine” | ferrebeekeeper, 2016]
Fig. 8 (bottom left) Diver and Nautilus. [Adapted from van de Week, 2016]
Diets
Cephalopods span across the depths of all five oceans, which also leads to differences in prey types. In other creatures, such differences lead to different mouth mechanisms — sharks do not eat the same way clownfish do, and parrots snack differently than hummingbirds. Yet somehow, the cephalopod beak serves a range of animals with minimal modifications to the base structure.
Here is a quick overview of the types of prey cephalopods eat, to demonstrate just how wide-ranging the beak needs to be. Starting with the biggest, giant squids prey on anything from small crustaceans to sharks. They have also been known to put up a pretty good fight with smaller whales, and even sperm whales, who will in turn hunt them when they get big enough. On the other hand, the squid Idiosepius (the smallest cephalopod, seen in Fig. 9) is limited to snacking on crab crumbs and worms (Fig.10).
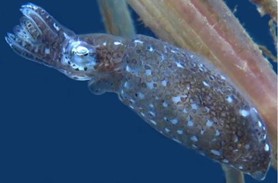
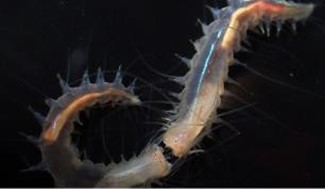
Octopuses, like all cephalopods, are carnivores who enjoy all types of meat and have even been known to catch birds who hunt fish in their proximity, as seen in Fig. 11.

Most octopuses prefer their privacy though, and live-in dens on the ocean floor, hunting bottom dwelling crustaceans (“What's The Difference Between Squid and Octopus?” | Leisure Pro, 2018). The favorite food of the cuttlefish is shrimp, but they will eat whatever creatures they can get their hands on (“Cuttlefish eat less for lunch when they know there'll be shrimp for dinner” | University of Cambridge, 2020). Or rather, their tentacles (refer to Quong, 2014 in Appendix). Similar to cuttlefish, nautiluses are opportunistic feeders who will hunt on whatever is most accessible to them, other nautiluses and dead fish included (Fig. 12).
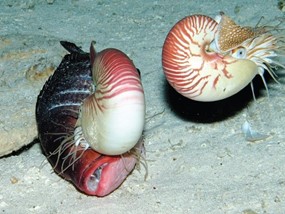
As well as size, environmental factors — including population size, competition, buoyancy requirements and location — contribute to the variance in diet of cephalopods (Navarro et al., 2014). However, most if not all cephalopods feast on marine crustaceans (Fig. 13) or shellfish, as they contain high levels of lipids and copper, which are both essential to the growth and survival of all cephalopods (Villanueva et al., 2017). Most only live for one or two years (Creighton, 2014), and in that time these active, fast-growing predators grow from tiny to whatever fate has in store, which can be quite large. Thus, it comes as no surprise that cephalopods have extremely high metabolic rates requiring plenty of proteins and other macro and micronutrients (Navarro et al., 2014). From worms to whales, these seemingly adorable creatures are not picky. They are feisty though and will even eat their own kind if challenged – or simply if they get the chance.
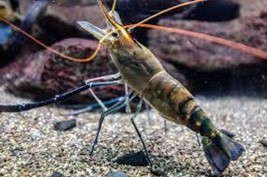
Strategies
Cephalopods are incredibly intelligent, with really strong memory, problem-solving and learning abilities (Ikeda, 2009). They are highly skilled hunters, using diverse feeding behaviors and hunting techniques to spot, trap and kill their prey. Their arsenal of hunting styles includes baiting, stalking, ambushing (refer to Indrisie, 2015 in Appendix), analytical maneuvering, camouflaging (“OCTOPUS Camouflage | Changes color, texture and shape” | Dive&Discover | YouTube, 2016) and sneak attacks (“Cephalopods On The Prowl!” | Monterey Bay Aquarium | YouTube, 2016). Cuttlefish even use their bioluminescence to hypnotize their prey before attacking (Fig. 14), and some octopuses pull shells towards them and stick their eyes out, waiting – and then strike (that is what is going on in Fig. 15). Each of these techniques has been tweaked over hundreds of millions of years, and a commonality among all is speed. Cephalopods themselves are hunted by many, including their own kind, as mentioned above. It is critical that they eat their meals quickly for their own protection. Thus, their hard, strong, decently large beaks are perfect for this: they can bite (or pierce) through and swallow whatever part of the prey is in front of them without needing to section it or take slow nibbles.
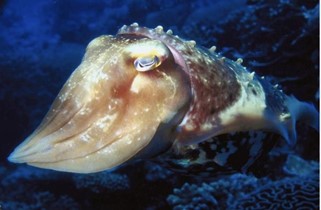
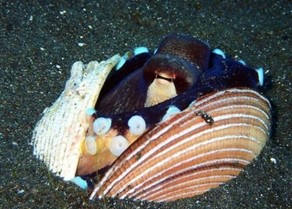
The Beak
Shape and Use
When a cephalopod eats, it catches its prey and uses its arms and tentacles to bring it towards the deadly device. Whether shelled or fleshy, the hard, sharp beak crushes/snaps/tears into the food, sometimes with the help of paralyzing toxins or a pick-like tongue. Cephalopods are pretty good at killing their prey quickly, which greatly simplifies feeding from then on. After that, the animal can chomp at its own pace (but not too slowly as life in the ocean is rarely leisurely) — with the beak serving as a dual knife-spoon combo, and the tentacles to aid with positioning (Villaneuva et al., 2017).
One mandible is thinner and sharper than the other, and tucks underneath when they overlap. The top and bottom segments of the beak are embedded in an extremely strong muscle tissue that forms a buccal mass as seen in Fig. 16. Cephalopods do not have jawbones, which means the beak is solely supported by the tissues of these dense muscles. They also control its movement (Boatman, 2014).
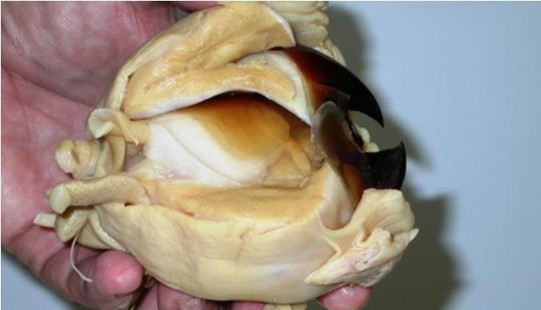
In general, the cephalopod beak is composed of an inner component, called the inner beak or wall, and an outer component, called the outer beak or the hood (Fig. 17). A long, slender strap called the bridge joins these two together with the help of the shoulder. The structure changes a bit from species to species, but the variations remain minimal overall.
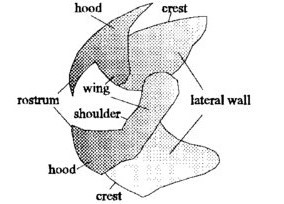
The differences are mainly related to the size and toughness of the prey consumed by each species. Cephalopods that feed on larger and/or tougher food tend to have larger and/or harder beak. The change in size is primarily along the sides of the beak, as that part is related to muscle attachment (a stronger animal has bigger, stronger muscles and needs a bigger beak to make use of them), while the change in hardness is in the rostrum — the sharp tip piece (Franco-Santos et al., 2013). Overall, they remain essentially the same in structure and in usage, despite the great variability of lifestyles touched upon above.
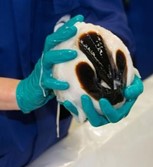
For a number of cephalopod species, studies have modelled the relationship between the size of different parts of the beak and the size of the individual. To do so, they used the remains of cephalopods that were still in relatively good condition. The purpose of establishing an identification key is to identify cephalopods, as well as estimate their size and biomass, based on the condition in which they are normally found: digested in a predator's stomach with only the beak remaining. These models generally study the mantle length as a measure of size of the individual and connect it to the rostral lengths of both the upper and lower beak. Although certain parts of the beak correlate as much if not more with mantle length, more data exists about rostral length in most species, and the rostrum is more resistant to digestion than other parts (Wolff, 1984).
In the Southern Ocean squid Psychroteuthis glacialis, for the plot of the lower rostral length (LRL) as the independent variable and the mantle length (ML) as the dependent variable (values in mm), the best fitted curve was a non-linear third order polynomial (Gröger et al., 2000):
ML = 50.6895 LRL – 8.6008
LRL2 + 1.0823
LRL3 – 8.7019 (1)
As for the relationship between the lower rostral length and the wet body mass (BM), the ln values of both variables were plotted against one another (values in mm), and the curve was linearized for the following function (Gröger et al., 2000):
ln(BM) = 0.3422 + 2.1380 ln(LRL) + 0.2214 ln(LRL)3 (2)
A similar study considered the beak of the deepwater squid Moroteuthis ingens. Due to sexual dimorphism in body size but not in beak size in this species, separate regression equations were determined for males and females (values in mm) (Jackson, 1995):
Males: ML = 24.40 LRL + 98.59 (3)
Females: ML = 44.36 LRL – 27.84 (4)
Similar relationships can be found for several other species. Unfortunately, there is no overarching rule to apply to cephalopods in general yet. This is the case for most of the field's research — data is quite limited and the few areas that have been looked into remain to be explored in much more depth.
Gradient
What is truly fascinating here is not the shape and multifunctionality, but the structure and what it allows. These animals are applying hard forces against sharp surfaces to break through the prey (like the cuttlefish biting through a crab in Fig. 19). How are they not cutting through their own remarkably gelatinous selves in the process? If their soft bodies were connected to the beak with no transition, when cephalopods tried to dig into their prey, the hard beak would rip through the surrounding soft tissue, doing as much damage to the hunter as to the prey through the equal and opposite reaction (Yong, 2008). The key to causing the amount of damage they can without harming themselves with each bite is a gradient of stiffness.
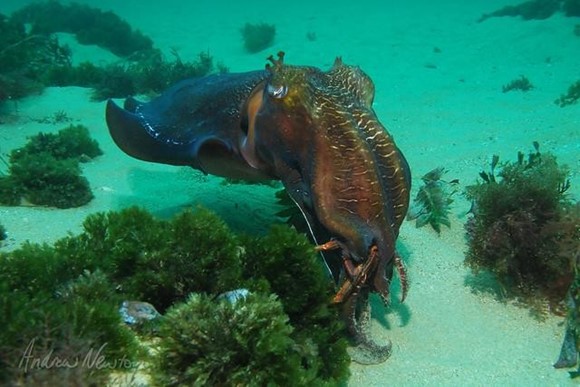
The cephalopod beak is composed of a tissue gradient. This is a gradient in both composition and mechanical properties. The strongest and hardest part of the beak is its tip. From there, the beak becomes progressively softer and more compliant, until it reaches the muscle attachments (Boatman, 2014). This gradient allows the beak to accommodate for large stresses when they bite into prey. The benefits of a mechanical gradient include increased distribution of interfacial mechanical and thermal stress, improvements in bonding of components with mismatched mechanical components, reduced contact deformation and damage, and the gradient makes it harder to fracture the graded material (Fox et al., 2013), which in this case, is the beak.
A very detailed study on the transition from soft to compliant materials in squid beaks focused on the Humboldt squid (Miserez et al., “The Transition from Stiff to Compliant Materials in Squid Beaks”). This is a particularly frightening yet interesting specimen. The Humboldt squid, pictured in Fig. 20, is also known as jumbo squid, or red devil. The nickname ‘jumbo' comes from its size: it has a 1.2-meter body with an added meter of tentacles and weighs over 50 kg (“Humboldt Squid” | Oceana Canada, n.d.). The nickname ‘red devil' comes from the fact that they flash red and white when caught by fishers. In fact, they can display at least 28 colors and flashing patterns that could have specific meanings and might even serve somewhat as a language between Humboldt squid kin (“Humboldt Squid” | Oceana Canada, n.d.). More on target, another magnificent feature of the Humboldt squid is its powerful beak, which is known to sever the spinal cords of their prey, paralyzing them for easier feeding (Miserez et al., “The Transition from Stiff to Compliant Materials in Squid Beaks”). It bites through skin and skull without differentiation, and their bitesize is about the size of one's fist (Cassell, 2005). The beak tip of the Humboldt squid is among the hardest and stiffest biomaterials out there, yet it transitions smoothly right into the soft muscular tissue it is embedded in (Miserez et al., “The Transition from Stiff to Compliant Materials in Squid Beaks”). The key to understanding this gradient is understanding the composition of the beak at different points.
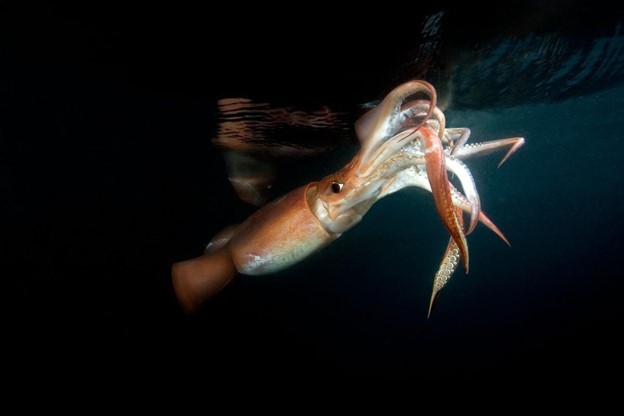
The beak has four key components: protein, water, pigment, and chitin, which is a polymer also found in insect exoskeletons (Yong, 2008). Humboldt squid beaks are the most extensively studied, so the following numbers were sourced from them, but the composition should be relatively similar across cephalopod species. In this case, chitin makes up 95 % of the beak's dry weight. The wing, seen in Fig. 21, is made up of about 70 % water, 25 % chitin, and 5 % protein. As you go to the tip of the beak, the water content decreases, chitin decreases, and the concentrations of protein and pigment increase.
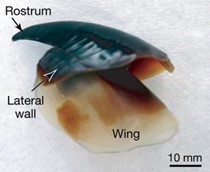
At the tip of the beak — the sharpest, hardest point — the water content reaches a minimum of 15 – 20 %, protein is at 60 %, pigment at 20 %, and chitin at less than 15 % (Miserez et al., “The Transition from Stiff to Compliant Materials in Squid Beaks”). It seems counterintuitive that chitin decreases as strength increases — hold on to that thought. One straightforward piece of information is that the gradient in hardness is accompanied by a pigmentation gradient that can be seen in Fig. 22 (Miserez et al., “The Transition from Stiff to Compliant Materials in Squid Beaks”). The darkest sections of the beak are an impressive 100 times stiffer than the lightest sections (Yong, 2008).
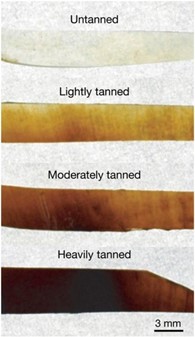
When the beak goes through an alkaline peroxidation — a process which removes all proteins and pigments — it reveals a scaffold of fine, 30 nanometer-wide, interconnected chitin fibers (Fig. 23) that maintain the beak shape (Miserez et al., “The Transition from Stiff to Compliant Materials in Squid Beaks”).
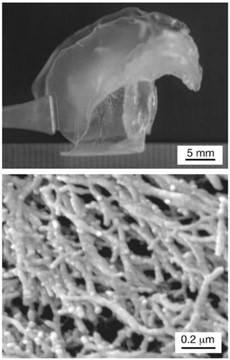
This chitin scaffold has very little structural integrity. A measurement that was used to determine this is the Young's modulus. Young's modulus describes the ability of a material to withstand changes in length when under tension or compression, and it is calculated by Eq. 7 (Editors of Encyclopedia Britannica, “Young's modulus”):
stress= {F\over A}
(5)
strain = {L_0 \over A(L-L_0)}
(6)
Young's \space Modulus = {stress\over strain}={FL_0\over A(L-L_0)}
(7)
where F is the tensile force, A is the cross-sectional area, L0 is the original length and L is the length after F is applied (Editors of Encyclopedia Britannica, “Young's modulus”).
In other words, Young's modulus is a measure of the material's stiffness. In the normal Humboldt squid beak, the Young's modulus, or stiffness, increases dramatically as pigmentation increases, from about 0.05 GPa to 5 GPa. However, after the beak goes through alkaline peroxidation, the Young's modulus drops to 0.03 GPa everywhere. This means that chitin is not what gives the beak its stiffness (Miserez et al., “The Transition from Stiff to Compliant Materials in Squid Beaks”).
In these beaks, stiffness decreases as chitin content increases, but chitin fibers are among the stiffest polysaccharides, and a go-to in the animal kingdom for building hard structures. So how can this be? Fascinatingly, it is because cephalopods have found something better. Not a material per se, but a weave: an extensive network of protein crosslinks between the pigment and the protein (Yong, 2008), neither particularly strong on their own. Yet, together, they contribute to the stiffness of one of the stiffest biomaterials in existence. At the same time, while pigment concentrations decrease moving towards the base of the beak, water and chitin concentrations increase, with water increasing three times faster. Thus, chitin is not a star player in this structure; it is overpowered, and its role is limited to giving shape. Hydration results in a more compliant material, and because water is increasing at a much greater rate, it plays a greater role than chitin in the stiffness gradient (Miserez et al., “The Transition from Stiff to Compliant Materials in Squid Beaks”). Water is a crucial ingredient in the cephalopod beak because it is what gives the beak its gradual compliance, making sure the base of the beak is soft and mechanically matched to the muscle it is embedded in (Yong, 2008). Because of the mechanical properties that result from the different compositions of the different parts of the beak, the beak tip is incredibly hard for tearing or breaking into prey's defenses, while the soft wing performs the remarkable task of insulating the buccal mass from the high interfacial stresses generated at the beak tip during feeding (Fox et al., 2013).
Structure
The cephalopod beak is made out of chitin fibers, composite proteins, and varying degrees of hydration which greatly contribute to its stiffness gradient (Miserez et al., “Cross-linking chemistry of squid beak”). The proteins in the Humboldt squid beak are rich in the amino acids known as histidine and a modified tyrosine called Dopa. These two amino acids bond with each other and create an extensive network of protein crosslinks that account for the stiffness of the beak and the dark color it exhibits (Yong, 2008). The increasing occurrence of these His-dopa cross-links and the cross-links of the multimers that make up the tanned pigment are likely to reduce solvation (Miserez et al., “The Transition from Stiff to Compliant Materials in Squid Beaks”). They do this by making a hydrophobic “coating” around the chitin fibers, thus preventing water absorption and consequently, beak softness. This is why the water content is so much lower at the tip of the beak (Miserez et al., “The Transition from Stiff to Compliant Materials in Squid Beaks”).
The way crosslinking allows cephalopods' beaks to achieve such hardness and durability is understood, but more research is required to understand the variations that occur between species, as well as the fine detail concerning protein-pigment arrangements that would allow this feature to be precisely mimicked.
Early Development and Growth
Cephalopods' beaks grow for the entirety of their lifetime (Dilly and Nixon, 1976), along with the rest of the animal. Yet the most significant and noticeable changes occur early on in their development and beginning in embryos. In Octopus vulgaris, the beak becomes significant enough that it can be removed from a specimen and studied towards the end of organogenesis (i.e., the formation of internal organs). More specifically, it can be studied at the same time in the animal's growth as when the mantle covers the gills (Armelloni et al., 2020; Deryckere et al., 2020).
Between the point when the beak can be examined and the developmental stage characterized by the internalization of the mouth (Deryckere et al., 2020), the upper jaw is very basic: its hood is not yet present, and teeth start to appear but lack pigmentation. Yes, teeth: serrated edges along the beak give baby cephalopods a boost during the time where they need to feed but do not have much strength in the beak yet. The beginning of dentition is accompanied by the development of the proteins that will be involved in the hardening and pigmentation of the beak (Armelloni et al., 2020). At the end of organogenesis and the beginning of an octopus' growth, a layer made of these proteins forms over the teeth (Fig. 24) to strengthen the beak and to form the shoulder and the hood of the beak (Deryckere et al., 2020).
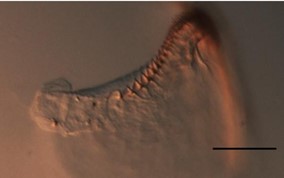
Just before the octopus hatches, the teeth's pigmentation appears, and the teeth keep growing and forming a protrusion (Armelloni et al., 2020) (Fig. 25). Once the paralarva — the young of the cephalopod — hatches, it starts using its beak, which keeps growing, and its teeth erode. This leads to the teeth forming a smooth, sharp apparatus: the rostrum – which looks much more like what one would expect on a beak (Franco-Santos et al., 2014).
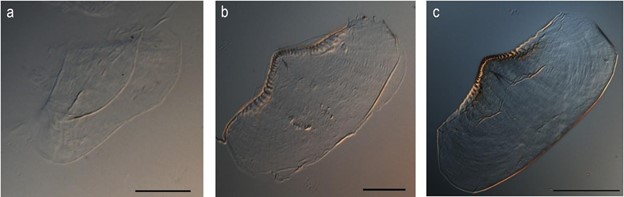
Though some cephalopods bear the classic beak model their whole life, many do have teeth early in their development. One such example is the Octopus vulgaris: as a paralarva, it has teeth on its upper jaw and its lower jaw, which it uses to grip its prey while it delivers digestive enzymes for external pre-digestion (Franco-Santos et al., 2014). Other species like Illex argentinus only have teeth in their lower beak, not both. This makes it much more difficult to bite into prey, but it has been suggested that these species use other parts of the beak along with their teeth to hold the prey in place. Eventually, both these species' teeth disappear and form the rostrum, the pointy bit of the beak. This coincides with the development of the cephalopod's limbs, which it can now use to hold prey as it bites into it with its beak (Franco-Santos and Vidal, 2020) so the teeth are no longer as useful.
At different stages of the octopus' development, certain parts of it grow at different rates, reflecting its particular functional needs. Between the late stages of organogenesis and the early stages of growth, the growth rate of the front of the beak decreases. Instead, this area focuses on reinforcement and hardening because it is used to slice and grab items. As for the back of the beak, it grows consistently at both stages of development. The sides of the beak also maintain a stable rate because their size is related to the size of the food ingested, which increases as the animal does. In addition, they do not need to harden like the front because their role is muscular attachment (Armelloni et al., 2020).
After the cephalopods hatch, their beaks keep growing. As the individual grows, it goes through a quick growth of the hood and lateral walls of the beak. As the animal increases in size, it can ingest larger items of food, which is needed as the animal's dietary needs increase with its body size. Similarly, a cephalopod that feeds on harder prey usually needs a more robust beak. For this reason, as cephalopods grow, they generally have more pigmented beaks, which are associated with stronger beaks, as discussed above. These features vary during an individual's lifetime, but also from one species to another based on their respective diets (Franco-Santos et al., 2014). For example, the Vampyroteuthis infernalis feeds on soft prey so they do not require beaks as hard as the Illex argentinus, whose diet includes harder prey like crustaceans and fish (Mouat et al., 2001). All in all, the cephalopod's beak goes through drastic changes during its lifetime to keep up with the animal's dietary habits and needs.
Applications
These beaks are incredible. But why does it matter? People can make use of understanding the cephalopod beak in a variety of concrete ways. Namely, applications in the medical field, principles that can go towards material engineering and data for studying the ocean's health.
Prosthetics
Unfortunately, the interphase between skin and a prosthetic limb can often see complications. In fact, skin problems are experienced by 75 % of amputees using a lower-limb prosthesis. Compared to the general population, amputees experience nearly 65 % more dermatological complaints (Highsmith et al., 2011). Prostheses introduce mechanical and thermal conditions the body is not used to. This can traumatize tissue through excessive tension, friction, heat, and pressure. With amputation, pressure-distributing anatomy (like the fat pad of the heel) could be missing or altered. The prosthesis must then be placed in an area that is not well suited for weight bearing. This can result in accelerated skin breakdown and pressure sores, especially when the socket is improperly placed (Highsmith et al., 2011).
Cephalopods have an incredibly robust way of mechanically matching the extremely stiff beak tip to their soft bodies. The stiffness gradient of their beak protects them from receiving harm every time they feed. Considering lower-limb prosthetics, the amputee's stump is under unusual pressure every time they take a step or even when they are standing still. A component of the prosthetic limb that makes contact with the amputee stump and that acts as a graded interphase could decrease the occurrence of pressure sores and other issues that result from the poor mechanical matching of the stump and the prosthetic limb. The gradient of this contact piece could be established with a similar combination of ingredients as that seen in the Humboldt squid beak (Highsmith et al., 2011).
Grips
Similarly, any surface that people apply pressure to when gripping could benefit from a gradient to ease that intensity. This includes wheelbarrows, paddles, handles on suitcases, shovels — anything that gives blisters to sensitive skin just trying to hold on. Rubber is often used, but a gradient system would be better still. Adding to that, the Humboldt squid could inspire environmentally friendly synthesis of polymer based composite materials such as the ones just described, as the Humboldt squid develops its beak in ambient sea water, and is wholly nontoxic (Miserez et al., “Cross-linking Chemistry of Squid Beak”).
Blades
The cephalopod beak is incredible beyond the smoothly transitioning gradient; the hard tip is a marvel in its own right. In fact, the beak of the jumbo squid is at least twice as hard and stiff as engineered organic materials, and it resists plastic deformation more effectively than almost all metals and polymers (Miserez et al., “Jumbo squid beaks”). The applications for materials so strongly resistant to deformation are countless. Figuring out how to imitate the crosslink patterns could be highly profitable, and serve in fabricating knives, shovels, drills, car bumpers, helmets, etc.
Robotics
Oftentimes in materials engineering and product fabrication, designs used are inspired by nature. Cephalopods, being so adaptable, are ideal subjects of study, especially when it comes to their unique structure. The hardness gradient that exists from the tip of their beak to its integration into their soft fleshy bodies is highly impressive. Developing an understanding of exactly how the gradient functions, could lead to the reproduction of these technologies in things such as robot design. A group of researchers developing robots are hopeful to produce robots which allow for safer human interactions by using softer materials and systems. However, they are presently struggling due to complications at the interfaces between rigid and soft components. This is where a firmness gradient comes in handy, by strengthening the robot's frame, specifically its transition between interior and exterior materials, so that any impacts the legs receive will not cause severe damage to the robot's inner mechanics. The robot seen in Fig. 26 is a jumping robot which uses inflatable legs to hop off the ground. The legs are inflated by what is known as an explosive actuator, which is just a fancy name for a pump, which fills the legs with oxygen and butane, then emits a spark, propelling the robot into the air. As combustion begins, there is a large increase in pressure which activates the movement of the robot, then as the gasses burn, the pressure decreases and the legs deflate (Bartlett et al., 2020). Force dissipation upon landing is clearly shown in Fig. 27, proving the gradient material is best at absorbing the impact more moderately. The gradient composite material connects the robot's soft shell (including said legs) to the core and its explosive actuator, lowering the risk of destroying its complex inner workings upon landing — just like how the contact between beak and meal is not harmful to cephalopods.
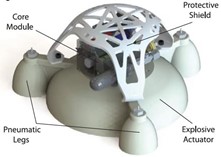
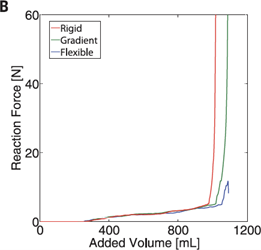
Conclusion
To conclude, cephalopods are truly marvelous creatures — even the more primitive nautilus. Their whole bodies are magnificently adapted to their needs and their beaks are a beautiful example of the brilliance of nature, given that the mother herself has been perfecting this particular project for hundreds of millions of years. In some ways, it could be suggested that cephalopods have evolved to best utilize their beak, rather than it evolving to suit them better (though certainly the tweaking between species is an indication that the adaptations go both ways).
Scientists are still far from understanding the complexities behind cephalopod behavior and thoughts and have only recently begun looking into the engineering behind their beak. There is still much to learn, but already it seems there is incredible potential to work with these discoveries.
Appendix
“Cephalopods On The Prowl!.”, 22 Jun 2016, Monterey Bay Aquarium, YouTube, https://www.youtube.com/watch?v=j8hfiaKAWgg.
“Cuttlefish eats fish alive.”, 26 Dec 2014, Pang Quong, YouTube, https://www.youtube.com/watch?v=MUCduZyCHes.
“OCTOPUS Camouflage | Changes color, texture and shape.”, 17 Jul 2016, Dive&Discover, YouTube, https://www.youtube.com/watch?v=XocHDvHlcJM.
“Octopus gets crabby in Yallingup.”, 18 Feb 2015, Porsche Indrisie, YouTube, https://www.youtube.com/watch?v=F5fZu-1bt6Y.
“Octopus Houdini.”, 15 Feb 2011, Zena Cardman, YouTube, https://www.youtube.com/watch?v=9yHIsQhVxGM.
References
Aquarium, M. B. (n.d.). Chambered nautilus. Retrieved from https://www.montereybayaquarium.org/animals/animals-a-to-z/chambered-nautilus/
Armelloni, E. N., Lago-Rouco, M. J., Bartolomé, A., Felipe, B. C., Almansa, E., & Perales-Raya, C. (2020). Exploring the embryonic development of upper beak in Octopus vulgaris Cuvier, 1797: New findings and implications for age estimation. Fisheries Research, 221, 105375. doi:10.1016/j.fishres.2019.105375
Bartlett, N. W., Tolley, M. T., Overvelde, J. T. B., Weaver, J. C., Mosadegh, B., Bertoldi, K., . . . Wood, R. J. (2015). A 3D-printed, functionally graded soft robot powered by combustion. Science, 349(6244), 161-165. Retrieved from https://science.sciencemag.org/content/sci/349/6244/161.full.pdf
Boatman, L. (2014). Crystallography in invertebrates: The cephalopod beak! Retrieved from https://nmnh.typepad.com/no_bones/2014/07/crystallography-in-invertebrates-the-cephalopod-beak.html
Britannica, E. o. E. (2019). Young's Modulus. Retrieved from https://www.britannica.com/science/Youngs-modulus
Caiger, P. (n.d.). A pop of red in the twilight zone. In: Woods Hole Oceanographic Institution. Retrieved from https://www.whoi.edu/multimedia/a-pop-of-red-in-the-twilight-zone/
Cambridge, U. o. (2020). Cuttlefish eat less for lunch when they know there'll be shrimp for dinner. Retrieved from https://www.sciencedaily.com/releases/2020/02/200208094304.htm
Canada, O. (n.d.). Humboldt Squid. Retrieved from https://oceana.ca/en/marine-life/cephalopods-crustaceans-other-shellfish/humboldt-squid
Cassell, S. (2005). Dancing With Demons – The Giant Humboldt Squid. Retrieved from https://www.deeperblue.com/dancing-with-demons/
Cato, J. (2019). What Kind of Water Does Shrimp Live In? Retrieved from https://sciencing.com/kind-water-shrimp-live-8419305.html
Creighton, J. (2014). Scientists Discover Longest-Living And Longest-Brooding Octopus: Guards Eggs For 4.5 Years. Retrieved from https://futurism.com/scientists-discover-longest-living-and-longest-brooding-octopus-guards-eggs-for-4-5-years
Darling, D. (2016). cephalopod. Retrieved from https://www.daviddarling.info/encyclopedia/C/cephalopod.html
Deryckere, A., Styfhals, R., Vidal, E. A. G., Almansa, E., & Seuntjens, E. (2020). A practical staging atlas to study embryonic development of Octopus vulgaris under controlled laboratory conditions. BMC Developmental Biology, 20(1), 7. doi:10.1186/s12861-020-00212-6
Dictionary, C. (n.d., 2021). mollusk Retrieved from https://dictionary.cambridge.org/dictionary/english/mollusk
Divers, T. F. (2017). Coconut Octopus on Holiday in Lembeh. Retrieved from https://www.twofishdivers.com/2017/06/coconut-octopus-holiday-lembeh/
Dunstan, A. J., Ward, P. D., & Marshall, N. J. (2011). Vertical Distribution and Migration Patterns of Nautilus pompilius. PloS One, 6(2), e16311. doi:10.1371/journal.pone.0016311
ferrebeekeper. (2016). Octopus Valentine. Retrieved from https://ferrebeekeeper.wordpress.com/tag/octopus/
Fox, J. D., Capadona, J. R., Marasco, P. D., & Rowan, S. J. (2013). Bioinspired Water-Enhanced Mechanical Gradient Nanocomposite Films That Mimic the Architecture and Properties of the Squid Beak. Journal of the American Chemical Society, 135(13), 5167-5174. doi:10.1021/ja4002713
Franco-Santos, R. M., Iglesias, J., Domingues, P. M., & Vidal, E. A. G. (2014). Early beak development in Argonauta Nodosa and Octopus Vulgaris (Cephalopoda: Incirrata) paralarvae suggests adaptation to different feeding mechanisms. Hydrobiologia, 725(1), 69-83. doi:10.1007/s10750-013-1721-4
Franco-Santos, R. M., & Vidal, E. A. G. (2020). Tied hands: synchronism between beak development and feeding-related morphological changes in ommastrephid squid paralarvae. Hydrobiologia, 847(8), 1943-1960. doi:10.1007/s10750-020-04223-z
Gröger, J., Piatkowski, U., & Heinemann, H. (2000). Beak length analysis of the Southern Ocean squid Psychroteuthis glacialis (Cephalopoda: Psychroteuthidae) and its use for size and biomass estimation. Polar Biology, 23, 70-74.
Hall, D. (2018). Cephalopods. Retrieved from https://ocean.si.edu/ocean-life/invertebrates/cephalopods
Hanlon, R. T., & Messenger, J. B. (1996). Feeding and Foraging. In Cephalopod Behaviour (pp. 47): Cambridge University Press.
Harper, D. (Ed.) (n.d.) Online Ethymology Dictionary. Retrieved from https://www.etymonline.com/word/cephalopod
Harzhauser, M. (1999). Filling a Gap -Beaks and Hooks of Cenozoic Coleoids (Cephalopoda). Annalen des Naturhistorischen Museums in Wien. Serie A für Mineralogie und Petrographie, Geologie und Paläontologie, Anthropologie und Prähistorie.
Heke, N. (2014). The beak of the colossal squid. In: Museum of New Zealand. Retrieved from https://www.tepapa.govt.nz/discover-collections/read-watch-play/science/anatomy-colossal-squid/beak-colossal-squid
Izzotti, A. (n.d.). coconut octopus underwater macro portrait on sand. In (Vol. 4041 x 2694px). Adobe Stock Adobe Stock. Retrieved from https://stock.adobe.com/ca/search?filters%5Bcontent_type%3Aphoto%5D=1&filters%5Bcontent_type%3Aillustration%5D=1&filters%5Bcontent_type%3Azip_vector%5D=1&filters%5Bcontent_type%3Avideo%5D=1&filters%5Bcontent_type%3Atemplate%5D=1&filters%5Bcontent_type%3A3d%5D=1&filters%5Bcontent_type%3Aimage%5D=1&k=octopus+&order=relevance&safe_search=1&limit=100&search_page=1&search_type=usertyped&acp=&aco=octopus+&get_facets=0&asset_id=87066417
Jackman, R. (2019). Sea Star Anatomy 101. In S. stomach (Ed.), Getty Images. ToughtCo. : Kennedy, Jennifer. Retrieved from https://www.thoughtco.com/starfish-anatomy-2291457
Jackson, G. D. (1995). The use of beaks as tools for biomass estimation in the deepwater squid Moroteuthis ingens (Cephalopoda: Onychoteuthidae) in New Zealand waters. Polar Biology, 15(1), 9-14. doi:10.1007/BF00236118
Mayes, K. (2019). Watch a tiny worm make one of the loudest sounds in the ocean. Science doi:10.1126/science.aay6798
McGee, K. (2013). European Cuttlefish. In. Flickr. Retrieved from https://www.flickr.com/photos/zombyluvr/9524397006/
Miserez, A., Li, Y., Waite, J. H., & Zok, F. (2007). Jumbo squid beaks: Inspiration for design of robust organic composites. Acta Biomaterialia, 3(1), 139-149. doi:10.1016/j.actbio.2006.09.004
Miserez, A., Rubin, D., & Waite, J. H. (2010). Cross-linking Chemistry of Squid Beak *. Journal of Biological Chemistry, 285(49), 38115-38124. doi:10.1074/jbc.M110.161174
Miserez, A., Schneberk, T., Sun, C., Zok, F. W., & Waite, J. H. (2008). The Transition from Stiff to Compliant Materials in Squid Beaks. Science, 319(5871), 1816-1819. Retrieved from https://science.sciencemag.org/content/sci/319/5871/1816.full.pdf
Morneau, G. (2012). Who's up for lunch? A Gull-eating Octopus in Victoria, BC. In. BirdFellow Journal Nightingale, Ann.
Mouat, B., Collins, M. A., & Pompert, J. (2001). Patterns in the diet of Illex argentinus (Cephalopoda: Ommastrephidae) from the Falkland Islands jigging fishery. Impact of Cephalopods in the Food Chain and their interaction with the Environment, 52(1), 41-49. doi:10.1016/S0165-7836(01)00229-6
Navarro, J. C., Monroig, Ó., & Sykes, A. V. (2014). Nutrition as a Key Factor for Cephalopod Aquaculture. In J. Iglesias, L. Fuentes, & R. Villanueva (Eds.), Cephalopod Culture (pp. 77-95). Dordrecht: Springer Netherlands.
Newton, A. (2010). Cuttle eating a red bait crab. In. Flickr. Retrieved from https://www.flickr.com/photos/pandasimages/4545414370
Octolab.tv. (2019). 7 Small Octopus Species That You'll Love. Retrieved from https://octolab.tv/7-small-octopus-species-that-youll-love/
Pro, L. (2018). What's The Difference Between Squid and Octopus? Retrieved from https://www.leisurepro.com/blog/explore-the-blue/difference-between-squid-and-octopus/
Reid, A. (2018). New thumbnail-sized pygmy squid discovered in Australia. In: Mongabay. Retrieved from http://www.fao.org/3/a0150e/a0150e05.pdf
Reid, A., Jereb, P., & Roper, C. F. E. (2005). Chambered nautiluses and sepioids (Nautilidae, Sepiidae, Sepiolidae, Sepiadariidae, Idiosepiidae and Spirulidae). In Cephalopods of the world. An annotated and illustrated catalogue of cephalopod species known to date. (Vol. 1, pp. 57-152): Food and Agriculture Organization of the United Nations (FAO).
Roper, C. F. E., & Voss, G. L. (2020). Cephalopod. Retrieved from https://www.britannica.com/animal/cephalopod
Rubin, J. L. (2020). 15 Tiny Miracles Existing Every Day Right Here On Planet Earth. Retrieved from https://littlethings.com/lifestyle/tiny-miracles
Runck, A. (2018, 20 Dec 2018). Southern Pygmy Squid. Retrieved from https://australian.museum/learn/animals/molluscs/southern-pygmy-squid-idiosepius-notoides-berry-1921/
Team, A. N. (2020). Adaptive Camouflage Helps Blend Into the Environment. Retrieved from https://asknature.org/strategy/adaptive-camouflage-helps-blend-into-the-environment/
themindcircle. (n.d.). Ever heard that an octopus can squeeze through any hole that its beak can fit through? Here is all the proof you need. Retrieved from https://themindcircle.com/ever-heard-octopus-can-squeeze-hole-beak-can-fit-proof-need/
van de Week, Z. (2016, 14 Mar 2016). Z/V/D/W Vintage – Nautilus. Retrieved from https://zeediervandeweekredactie.wordpress.com/2016/03/14/zeedier-van-de-week-vintage-v/
Villanueva, R., Perricone, V., & Fiorito, G. (2017). Cephalopods as Predators: A Short Journey among Behavioral Flexibilities, Adaptions, and Feeding Habits. Frontiers in Physiology, 8(598). doi:10.3389/fphys.2017.00598
Weaver, T. (2012). Some Cephalopods: Inking About Squid Pens. Retrieved from https://naturalsciencesresearch.wordpress.com/2012/06/11/some-cephalopods-inking-about-squid-pens/
Wolff, G. A. (1984). Identification and estimation of size from the beaks of 18 species of cephalopods from the Pacific Ocean.
Yong, E. (2008). A Squid's Beak is a Marvel of Bioengineering Design. Retrieved from https://www.nationalgeographic.com/science/article/a-squids-beak-is-a-marvel-of-biological-engineering#close