Abstract
Birthing is the process that keeps animals involved within evolution. Nature encourages animals to reproduce and to allow their offspring to prolong the life of their species. These intricate processes of parturition and hatching are based on mathematical concepts such as feedback loops, where stimulus generates stimulus, and circadian rhythms. While creatures of nature tend to lie in a homeostatic state, there comes a time where certain mechanisms take over and become quite unregulated. This occurs in both viviparous and oviparous animals, which are animals that give birth and those that lay eggs, respectively. During mammalian parturition, contraction induced oxytocin release creates a positive feedback loop, whereas increase in cortisol in the fetus decreases the concentrations of other hormones creating a negative feedback loop. Furthermore, early embryonic developments and hatching in silkworms and fish display circadian rhythmic systems that are affected by light and temperature cycles of the surrounding environment. Circadian rhythms help ensure that physiological processes in the egg occur at appropriate times during the 24-h day/night cycle. These rhythms are generated and sustained at the cellular level by transcriptional-translational negative feedback loops that cycle daily and are entrained by environmental cues, including light and temperature.
Introduction
The birthing and hatching process in most creatures is characterized by the use of rhythms and patterns throughout. These mathematical processes are crucial to the functioning of these systems in animals, and allow for a birthing or hatching process without issue. Many animals employ feedback loops within the birthing process in order to regulate the hormones necessary to complete parturition. These feedback loops either allow for the constant increase of an event in a system, or a constant decrease. In the hatching process, feedback loops are also employed with respect to temperature of the environment and the light/dark cycle.
This paper will first discuss the use of feedback loops in parturition, specifically examining these processes in mammals. Positive feedback loops exist in birthing between the cervix and the hormone oxytocin. The pressure on the cervix induces a secretion of oxytocin, thus inducing more contractions, and therefore more oxytocin secretion (Russel et al., 2003). In other mammals such as the sheep, however, negative feedback loops also occur. Certain hormones such as leptin and cortisol are necessary in the sheep fetus. These hormones regulate each other, as the decrease in leptin concentration allows for an increase in the cortisol concentration (Yuen et al., 2004). The increase in cortisol also aids in inducing parturition (Wood & Keller-Wood, 2000).
Next, this paper will discuss the presence of hatching rhythms in silkworms and fish, specifically in terms of the effects that light and temperature have on these rhythms. Silkworm eggs have evolved to respond to light stimuli in order to regulate their hatching rhythms, based on the many interactions they have with their environment. More precisely, light stimuli affect the transcription levels and expression of different genes involved in the physical hatching process and the circadian oscillator, or circadian clocks, in silkworms, through an oscillating hatching rhythm and a negative feedback loop (Tao et al., 2017).
Fish have also adapted to periodic changes in their environment by evolving a circadian system that allows physiological processes and behavior in the egg to occur at specific conditions from anticipating external changes in light and water temperature (Mosser et al., 2019). In particular, the remarkably early and rapid development of the Senegalese sole circadian system has facilitated exploring the factors that control the onset of circadian clock function during embryogenesis and hatching. The impact of light and temperature at various regimes on the circadian cycle in the fish embryo soon after fertilization points to the mechanism of daily rhythms in embryonic development and hatching synchronized to certain photoperiods and surrounding temperatures (Villamizar et al., 2013).
Feedback Loops in Parturition
Oxytocin Positive Feedback Loops
As animals proceed through the birthing process, there are many mathematical theories and aspects that ensure a smooth and safe parturition. For viviparous animals, those that give birth to live young, an important positive feedback loop occurs.
A positive feedback loop happens when an initial stimulus prompts more of the initial stimulus, therefore leading to a never-ending loop of increased stimulation if left unregulated. Such a mechanism is present in the birthing process of many animals. A main component of parturition for many animals is uterine contractions. For the fetus to exit the mother’s reproductive organs, it must be pushed out through the uterus. This occurs through a repetitive biomechanical reconstruction of the muscles present in the uterus, primarily in the myometrium, which is a layer of muscular tissue in the uterine wall (Malik et al., 2020). These contractions are induced by levels of oxytocin in the mother’s body. Oxytocin (OT) is a peptide hormone that is produced in an animal’s hypothalamus, a small region at the base of the animal brain (Russel et al., 2003). During parturition, mammals such as rats experience increased activity of their oxytocin cells contrary to those not carrying a fetus. This phenomenon displays the nature of a positive feedback loop. Once the fetus begins to exit the uterus, there is an applied pressure on the cervix, the component of the uterus responsible for contractility. This active contact inhibits a burst of action potential, which induces the secretion of oxytocin in the hypothalamus, which encourages more contractions, which produces another burst and then another secretion (Russel et al., 2003). This loop continues until the fetus finally exits the mother (Fig. 1).
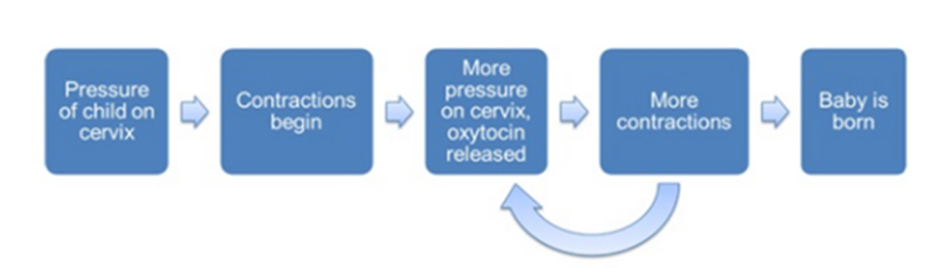
This model can also be expressed with the use of differential equations and rates of change. Researchers describe the oxytocin release as the firing rate, and this can be described graphically. The firing rate is the frequency at which an oxytocin cell propagates action potential, measured in spikes per unit time (Gerstner et al., 1997). Studies done on rats during parturition showed an evident increase in the firing rate of oxytocin pulses (Fig. 2). This increase demonstrates the developing positive feedback loop that induces contractions while the fetus is traveling through the uterus (Russel et al., 2003).

Effects of Cortisol and Leptin Hormones on the Birthing Process in Sheep
In sheep, there are a few hormones that are crucial to the success of the birthing process and delivery of a healthy offspring. In general, cortisol is thought to be the most important of theses. Cortisol is key during sheep pregnancy in the development and maturation of key organs within the fetus (Yuen et al., 2004). Cortisol is also very important in the beginning of the parturition process as it is crucial to its timing (Yuen et al., 2004). Leptin is another important hormone present during sheep parturition, and it plays a very different role. It acts in regulation of homeostasis, as well as reproductive functions (Yuen et al., 2004). Additionally, where fat is deposited in the fetus before birth, leptin is synthesized by adipose cells and circulated late in gestation (Yuen et al., 2004). The interactions between these hormones in the sheep during gestation and parturition lead to the development of negative feedback loops. This is when an initial stimulus is fed back and causes the stimulus to be reduced.
The ratio of concentrations of leptin to cortisol changes throughout the pregnancy in sheep. By the end of term–a few days before the birth–the leptin concentration decreases slowly in the fetus, whereas cortisol concentration increases rapidly. This is most noteworthy 10 days before labor begins (Yuen et al., 2004). This suggests a negative relationship between leptin and the adrenal gland (where cortisol is produced). It is hypothesized that the initial decrease of the leptin concentration in the fetus allows for a greater concentration of cortisol, which may further decrease the leptin concentration, acting in a negative feedback loop (Yuen et al., 2004).
The increase in cortisol is crucial to the end of the pregnancy. In one study where sheep fetuses were infused with leptin, which increased the concentration 4-5-fold, the normal increase of cortisol at the end of the term was suppressed, keeping the overall concentration low until hours before delivery (Fig. 3) (Yuen et al., 2004). Cortisol also influences the induction of the parturition process (Wood & Kellerwood, 1991). The increase of the concentration of cortisol in the fetal circulatory system close to delivery induces the secretion of other hormones, namely estrogen, and the increase of estrogen decreases the concentration of progesterone. This increase of the estrogen to progesterone ratio may help lead to labor (Fig. 4) (Wood & Kellerwood, 1991). Therefore, the increase in cortisol concentration due to a decrease of leptin concentration has other effects. It leads to the increase of estrogen concentration and a decrease of progesterone concentration. This chain reaction can then increase uterine contractions, and aid in initiating labor (Wood & Kellerwood, 1991). These two negative feedback loops, one of cortisol, and the other of estrogen, work together to lead to the beginning of parturition in sheep.


Another hormone that is present during sheep gestation is the adrenocorticotropic hormone (ACTH). This hormone is secreted from the pituitary gland and has been shown to be involved in the timing of the parturition process in sheep (Challis et al., 2000). ACTH and cortisol concentrations increase steadily in the sheep fetus during the gestation period, with a rise in ACTH concentration preceding the exponential rise in cortisol concentration later at the end of gestation (Fig. 5) (Challis et al., 2000). However, normally, an increase in ACTH concentration lowers the concentration of cortisol, and vice-versa (Challis et al., 2000). This would therefore induce a negative feedback loop, where the rise in concentration of one would lower the other and continue a chain reaction. This is hypothesized to be due to mechanisms present in the fetus before the birthing process. Glucocorticoid receptors are responsible for bonding to these cortisol molecules. These receptors become less expressed when the concentrations of both cortisol and ACTH increase, thus not being able to regulate and create that negative feedback loop resulting in one concentration increasing and another decreasing. (Challis et al., 2000). The prohibition of these feedback loops is therefore necessary to keep the fetus healthy and allows for a proper birthing process later once the fetus is developed and ready for parturition. Since both these hormones are necessary either for the timing of the birth (ACTH) or the development of the fetus and its organs (cortisol), it is important that negative feedback does not occur.
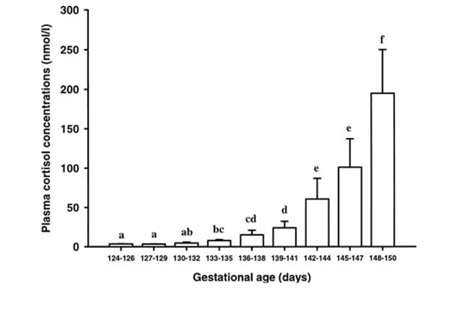
Effect of Light and Temperature on Hatching Rhythms
Hatching Rhythms of Silkworms
The Bombyx mori, more commonly known as the silkworm, is a Lepidoptera species usually associated with the production of silk. This insect is one of many that has hatching rhythms which are affected by the time of day or night. In silkworms, the light/dark cycle is the most important factor which affects hatching rhythms of their eggs, specifically by creating a negative feedback loop of the circadian oscillator during the embryonic stage of development (Tao et al., 2017). Hatching rhythms play a key part in the survival of the vulnerable larvae of this species since these rhythms can greatly affect the degree of survival of larvae depending on when hatching is set to occur.
Under the natural rhythmic cycle of light and dark in the environment, the hatching rhythms of silkworms oscillate in time with the cycle of light. Furthermore, the transcription and expression of genes involved in the regulation of the circadian oscillator exhibit a negative feedback loop. However, when the cycle of light and dark of the environment is lost, the hatching rhythms of silkworm are greatly disrupted. This concept was explored in the study done by Tao et al. (2017) in which four different regimes of ambient light were tested on groups of silkworm eggs. These regimes were 12-hour light: 12-hour darkness (12L:12D), 12-hour darkness: 12-hour light (12D:12L), constant light (LL), and constant darkness (DD). The purpose of including two identical light/darkness regimes with inversed order was to show that the hatching rhythms would be dependent on when the light was turned on, regardless of when the study began. The eggs were analyzed the day before hatching and the day of hatching. Specifically, the effect of light and dark cycles was analyzed by looking at the transcription levels of chitinase5 (Cht5) and hatching enzyme-like (Hel) genes, and the enzymatic activities of their gene products, CHT and HEL, respectively. These genes are essential components in the process in which insects dissolve and soften the eggshell during hatching with digestive juices (Tao et al., 2017). In addition, this study examined the expression of cryptochrome 1 (Cry1), cryptochrome 2 (Cry2), period (Per), and timeless (Tim) genes, which are central clock genes involved in the establishment of the negative feedback loop of circadian rhythms.
The Zeitgeber time zero hour (ZT0) was established as the time at which light was turned on, and ZT12 was when the light was turned off, in both light/darkness (LD) regimes (Tao et al. 2017). At ZT0 in the 12L:12D regime, hatching rates experienced a peak on the day of hatching, day 12, and the remaining eggs experienced a hatching peak at the ZT0 of the following day, day 13. In the inverted light-darkness regime, 12D:12L, the hatching peak was also experienced at ZT0 of day 12. Thus, regardless of whether the light cycle started with light or darkness, the hatching rates of silkworm eggs peaked at times when the light was turned on (Fig. 6).
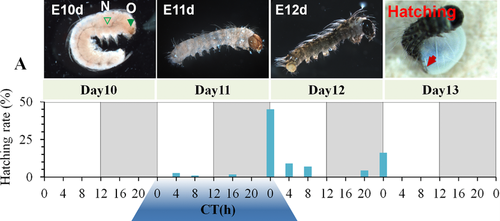
When looking at the transcription levels of Cht5 and Hel genes, they experienced a significant peak in transcription right before and 8 hours before hatching, respectively. When analyzing the activity levels of the CHT and HEL proteins, they were shown to have increased activities from ZT16 to ZT24, meaning from late at night to dawn, right before hatching (Fig. 7). As with the hatching rates, the peaks in CHT and HEL activity once again occurred regardless of the LD regime imposed on the group, indicating that the light stimulus induced a specific hatching rhythm in the silkworm eggs centered around the turning on of the light.

In terms of the transcription levels of Cry1, Cry2, and Tim during the LD regimes, these genes were shown to have synchronous rhythms of expression with peaks at ZT0, while PER had a rhythm anti to the other clock genes, with peaks at Z12. In addition, there was an accumulation of the gene products for these four clock genes in the last phase of development before hatching, and after reaching their maximal levels right before egg hatching, they then rapidly returned to their levels prior to hatching. As these genes are specifically related to the circadian oscillator in silkworms, these results showed their involvement in the circadian rhythm negative feedback cascade (Tao et al., 2017).
In contrast to the LD regimes, the LL and DD regimes showed a total disruption in the oscillating hatching rhythms previously described. Having constant light or constant dark caused the hatching rates to be spread out throughout the day, so that no concrete peaks of hatching occurred (Fig. 8).
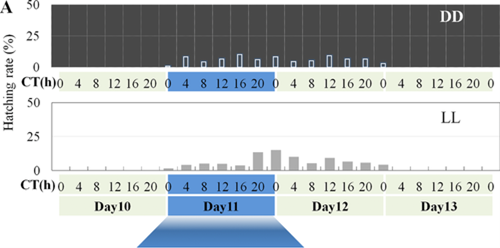
Similar to the hatching rates, the levels of transcription of the Cht5 and Hel genes did not show defined peaks of expression right before ZT0, unlike the genes during the LD regimes (Fig. 9). Furthermore, the transcription levels of the clock genes involved in the circadian rhythm negative feedback cascade showed changes in their expression. Specifically, under LL and DD conditions their amplitude and phase differed compared to what occurred during the LD regimes, becoming more irregular.
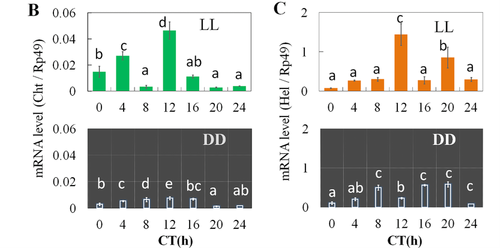
Thus, when analyzing the results of this study, it can be seen that the hatching rhythms of silkworms are highly dependent on regular light cycles in order to form a circadian rhythm negative feedback loop which results in an oscillating rhythm (Tao et al., 2017). The expression of genes directly related to the hatching process in silkworm occurs similarly to the rhythm of hatching behavior, thus acting as regulators for hatching (Tao et al., 2017). The disruption of these hatching rhythms in silkworms due to a change in external light stimuli would jeopardize the chance of survival of the vulnerable silkworm larvae.
Circadian Rhythms during Embryonic Development and Hatching in Senegalese Sole
During biological development, fish display rhythmic physiological processes that are shaped by daily changes in their surrounding environment (i.e., light and temperature cycles). These rhythms are generated and sustained at the cellular level by transcriptional-translational negative feedback loops that cycle daily and are entrained by environmental cues. Like most living organisms, fish have adapted to the natural periodic changes in their environment by evolving a circadian system, or biological clock that measures internal time and anticipates external events (Blanco-Vives et al., 2011). However, unlike land animals, fish inhabit a highly dynamic environment characterized by daily, lunar, and annual light cycles driven by the rotational movements relative to the Earth and sun (Zhdanova & Reebs, 2005). The Senegalese sole, a flatfish with a strictly nocturnal behavior, is among the countless fish that exhibit circadian rhythms.
In the study done by Villamizar et al. (2013), the fertilized eggs of Senegalese soles were exposed to three light regimes: 12 h of light and 12 h of darkness cycle (LD), continuous light (LL), or continuous dark (DD); and three temperature treatments: 18°C, 21°C, or 24°C. Three different stages were established as the evaluating parameters of embryo development. The stages within fish embryo development used to evaluate the effect of light and temperature conditioning on the Senegalese sole were divided based on hours post fertilization (hpf). Stage 1 represents the Dome stage when epiboly starts (~4 hpf), Stage 2 exhibits 8 somites in the embryo when the optic primordium has developed (~13 hpf), and Stage 3 exhibits 20 somites when the pigmentation begins and a median fin fold extends along the body of an embryo fish (~24-25 hpf).
In all three stages of embryonic development, Senegalese sole embryos reared in LD and LL demonstrated similar paces of development, whereas in DD conditions, there was a decrease in the rate of embryogenesis by an additional 8-10 h. Higher temperatures led to advanced embryo development, although at some sampling points no significant differences were found between 21°C and 24°C (Fig. 10).
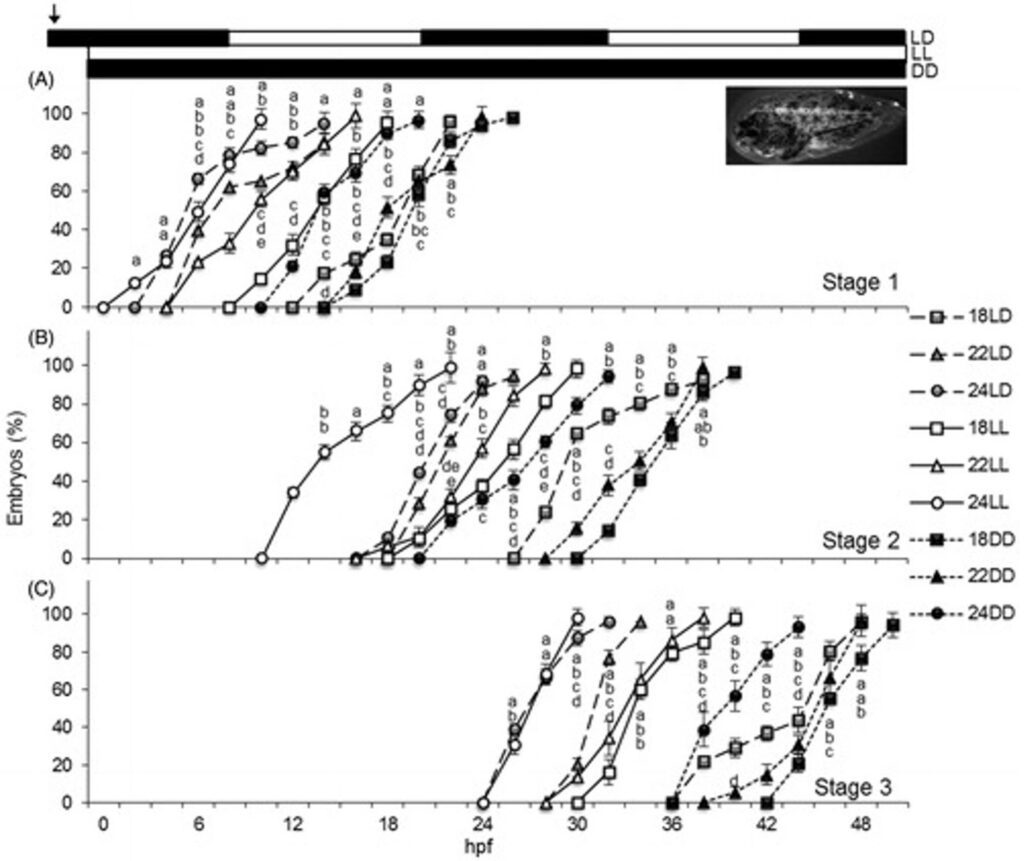
Corresponding to differences in the rate of embryonic development of Senegalese soles in various temperatures and LD regimes, hatching rhythms likewise differed among soles in varying regimes. For sole embryos in the 12:12 LD cycle at higher temperature of 22°C and 24°C, hatching was observed occurring earlier during the first dark phase (peaking at 28-30 hpf) (Fig. 11A). Meanwhile, the soles in 18°C hatched during the second night (peaking at 55 hpf). A specific nocturnal rhythmic pattern of hatching was observed with a single hatching peak per temperature group throughout the LD cycles.
For the other two light regimes, hatching was especially advanced in continuous light (LL) so that embryos hatched on the first subjective day in LL Fig. 11B), whereas hatching was delayed to the second day in continuous dark (DD) conditions (Fig. 11C). At 24°C hatching occurred earlier (peaking by 29 and 48 hpf in LL and DD, respectively), whereas at 22°C and 18°C hatching occurred later (peaking at 34–38 hpf in LL and 57–58 hpf in DD). In sole under LL conditions, embryonic development was faster and hatching bouts were grouped around the first subjective day, whereas in DD the development was slower and hatching occurred the second subjective day. Additionally, higher temperatures of each regime resulted in faster embryonic development and hatching.
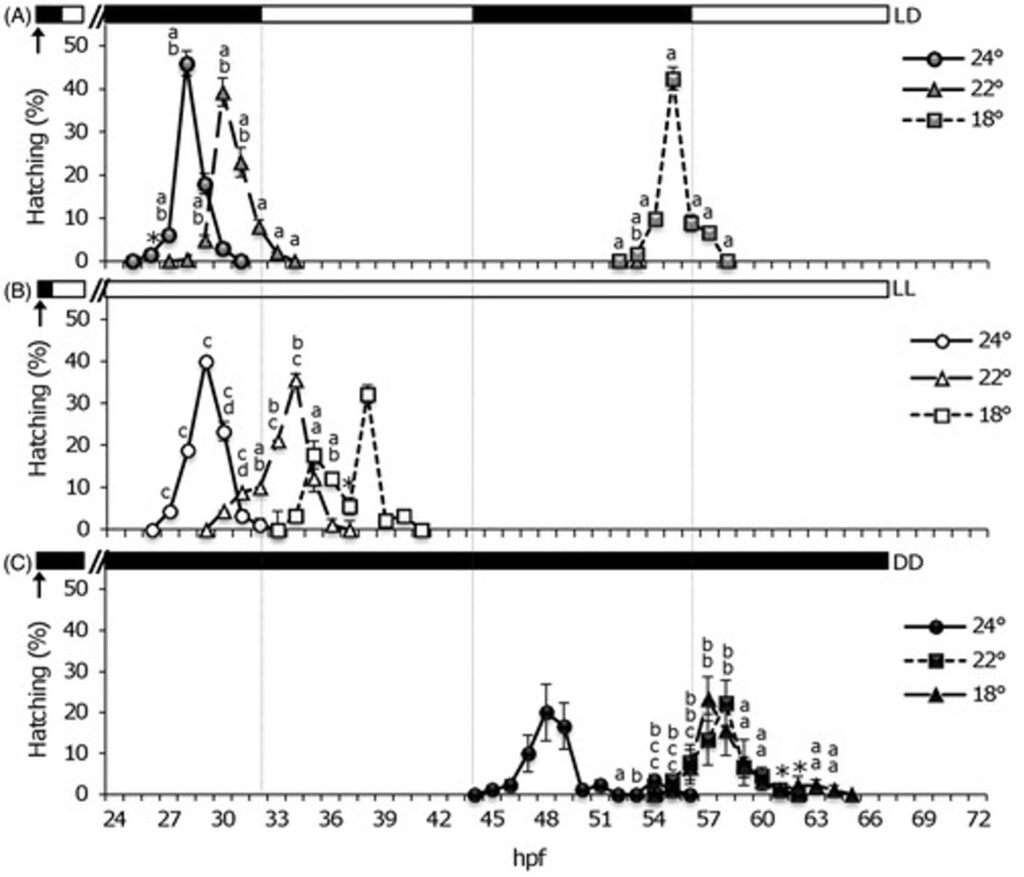
The results of embryogenesis and hatching rhythms expressed by the effects of daily photocycles and their interactions with temperature during early developmental stages points to the significance of circadian rhythms in Senegalese soles.
CONCLUSION
Many processes occurring in nature seem unorganized or random but are actually dependent on specific mathematical concepts, and the crucial life event of birth or hatching is no exception. In this case, the birthing and hatching of animals is greatly dependent on the existence of feedback loops, both positive and negative, as well as rhythmic cycles affected by environmental stimuli. When examining the birthing of mammals, it becomes clear that positive and negative feedback loops govern the induction of parturition through the regulation of specific hormones related to labor. More precisely, the secretion of oxytocin throughout parturition occurs in a positive feedback loop to induce contractions, while the regulatory relationship between the cortisol and leptin hormones exists as a negative feedback loop. In the eggs of oviparous animals, circadian rhythms and feedback loops affect and describe the hatching rhythms that occur in species such as the silkworm and the Senegalese sole. External environmental stimuli such as the cycle of light and dark as well as temperature affect these hatching rhythms and the transcription of certain genes involved in the establishment of a biological clock in embryos exhibit negative feedback loop behavior. These responses to external stimuli have evolved over time as a survival tactic for vulnerable eggs. Therefore, it is clear that mathematical processes play an essential role in the earliest stages of animal life.
References
Blanco-Vives, B., Aliaga-Guerrero, M., Cañavate, J. P., Muñoz-Cueto, J. A., & Sánchez-Vázquez, F. J. (2011). Does Lighting Manipulation During Incubation Affect Hatching Rhythms and Early Development of Sole? Chronobiology International, 28(4), 300-306. https://doi.org/10.3109/07420528.2011.560316
Challis, J. R. G., Matthews, S. G., Gibb, W., & Lye, S. J. (2000). Endocrine and Paracrine Regulation of Birth at Term and Preterm. Endocrine Reviews, 21(5), 514-550. https://doi.org/10.1210/edrv.21.5.0407
Gerstner, W., Kreiter, A. K., Markram, H., & Herz, A. V. M. (1997). Neural codes: Firing rates and beyond. Proceedings of the National Academy of Sciences, 94(24), 12740-12741. https://doi.org/10.1073/pnas.94.24.12740
Malik, M., Roh, M., & England, S. K. (2021). Uterine contractions in rodent models and humans. Acta Physiologica, 231(4). https://doi.org/10.1111/apha.13607
Mosser, E. A., Chiu, C. N., Tamai, T. K., Hirota, T., Li, S., Hui, M., Wang, A., Singh, C., Giovanni, A., Kay, S. A., & Prober, D. A. (2019). Identification of pathways that regulate circadian rhythms using a larval zebrafish small molecule screen. Scientific Reports, 9(1). https://doi.org/10.1038/s41598-019-48914-7
Russell, J. A., Leng, G., & Douglas, A. J. (2003). The magnocellular oxytocin system, the fount of maternity: adaptations in pregnancy. Frontiers in Neuroendocrinology, 24(1), 27-61. https://doi.org/https://doi.org/10.1016/S0091-3022(02)00104-8
Tao, H., Li, X., Qiu, J.-F., Liu, H.-J., Zhang, D.-Y., Chu, F., Sima, Y., & Xu, S.-Q. (2017). The light cycle controls the hatching rhythm in Bombyx mori via negative feedback loop of the circadian oscillator. Archives of Insect Biochemistry and Physiology, 96(2), e21408. https://doi.org/https://doi.org/10.1002/arch.21408
The Albert Team. (2020, June 1). Positive and Negative Feedback Loops in Biology. Albert. Retrieved November 28, 2021, from https://www.albert.io/blog/positive-negative-feedback-loops-biology
Villamizar, N., Blanco-Vives, B., Oliveira, C., Dinis, M. T., Di Rosa, V., Negrini, P., Bertolucci, C., & Sánchez-Vázquez, F. J. (2013). Circadian Rhythms of Embryonic Development and Hatching in Fish: A Comparative Study of Zebrafish (Diurnal), Senegalese Sole (Nocturnal), and Somalian Cavefish (Blind). Chronobiology International, 30(7), 889-900. https://doi.org/10.3109/07420528.2013.784772
Wood, C. E., & Keller-Wood, M. (1991). Induction of parturition by cortisol: effects on negative feedback sensitivity and plasma CRF. Journal of Developmental Physiology, 16(5), 287-292. https://www.ncbi.nlm.nih.gov/pubmed/1668556
Yuen, B. S. J., Owens, P. C., Symonds, M. E., Keisler, D. H., McFarlane, J. R., Kauter, K. G., & McMillen, I. C. (2004). Effects of Leptin on Fetal Plasma Adrenocorticotropic Hormone and Cortisol Concentrations and the Timing of Parturition in the Sheep1. Biology of Reproduction, 70(6), 1650-1657. https://doi.org/10.1095/biolreprod.103.025254
Zhdanova, I. V., & Reebs, S. G. (2005). Circadian Rhythms in Fish. In Fish Physiology (Vol. 24, pp. 197-238). Academic Press. https://doi.org/https://doi.org/10.1016/S1546-5098(05)24006-2