Mathematical Model Trends Between Longevity and Social Structure Dynamics in the Mammalian Class
Alex Butler, Sophie McLean, Alexandru Toma, Kevin Xu
Abstract
The mammalian class is home to many different unique species of animals, who have adapted to raise their young and prosper in countless manners. Bats, elephants, baboons, and even killer whales all strive to nurture their offspring and have developed many social dynamics to aid their survival and provide parental care. The vast array of population structures can be boiled down to several essential social characteristics, through which any mammal’s social lifestyle can be classified. Using researched mathematical models, several mammalian populations were studied to observe the relations and general trends between their given longevity and their social structures’ qualitative characteristics. Varying longevity patterns within mammalian social communities were observed when breaking down their social structure to study their primary units, tolerance, organization level, offspring membership, sex ratio, group size, seasonal cycling and group stability. These trends provide us with a greater understanding of how populations interact with their environments and other species, which is not only fascinating but may also be of great use in any social structure to improve individual health and longevity, and even in future studies of endangered or captive animals, to further understand their conditions and support their survival.
Introduction
Sociality is a term to describe how much the individuals of a species tend to associate themselves and their group with others of their kind. These can be in pairs, small, or large groups, they can be during the mating season only, they can have varying degrees of tolerance, or they can even differ in the level of effort and resources parents invest in raisingtheir offspring. In short, social structure dynamics vary immensely from species to species. Some studies have separated these distinctions into 8 dimensions: primary unit, tolerance, levels of organization, offspring membership, sex ratio, group size, seasonal cycling, and stability. There have been many cases that suggest that sociality may lead to greater longevity, such as with species that have “grandmothers” having longer lifespans and slowing the effects of senescence. As can be seen with the various dimensions of sociality, there are many factors that come into play that may affect longevity in a positive or negative way. Many different theories of ageing present contradicting elements that hold true on their own, therefore it might not be quite simple to find a clear and precise trend between sociality and longevity.
Trends between longevity, primary units and tolerance
Primary units in the animal kingdom can be defined by three categories: individual, pair/family, and group (Prox & Farine, 2020). Social species often experience lower rates of extrinsic mortality, due to reduced probability of being targeted by a predator, resource defense, greater efficiency in foraging, assistance in rearing or protecting young, among other factors (Lucas & Keller, 2020). In species with high rates of extrinsic mortality, the evolution of delayed senescence is not favored over other factors that may decrease extrinsic mortality at the expense of a long lifetime. For example, mammals that suffer high rates of extrinsic mortality such as mice do not need to evolve methods to combat cancer growth as they are less likely to experience senescence. However, in species with low rates of extrinsic mortality, animals that were usually able to survive into adulthood would then experience less senescence and have relatively long lifespans (Fig. 1).
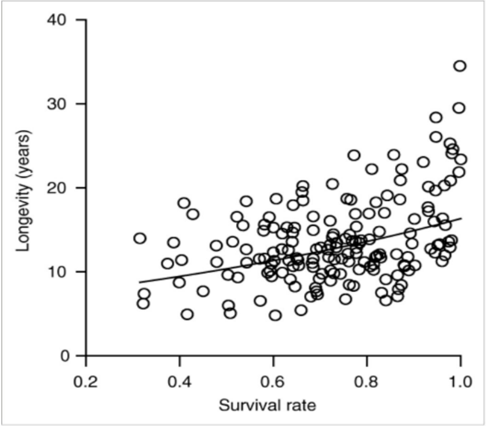
The converse may also be argued that longevity promotes more interspecific social interaction, especially familial groups. Increased longevity increases the population density, forcing young to remain near their kin, especially for territorial species. Proximity provides more opportunity for social reaction, so the species must learn to interact with family members, possibly resulting in the evolution of familial groups. A reinforcing cycle may form whereby longevity promotes social cooperation which in turn reduces extrinsic mortality, putting more pressure on the evolution of traits that delay senescence (Fig. 2).
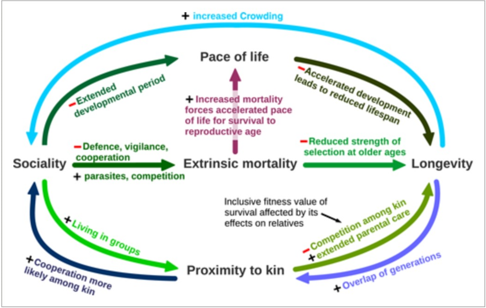
Many bird species have evolved to congregate in flocks to improve chances of survival through improved foraging and detection of predators (Beauchamp, 2021). Flocks may contain one species or multiple and may remain together year-round or temporarily; some may disperse during the breeding season in response to increased territorialism. In a comparative study involving the life span and flocking tendencies of 1146 bird species, it was found that flocking species will generally survive longer than solitary species (Fig. 3). On average, flocking behavior added approximately 3 percentage points to adult annual apparent survival, although this was not consistent across all species (such as Ca Charadrius alexandrinus, the English plover) (Beauchamp, 2021). Occasionally flocking species also showed higher survival rates than solitary species, however, the effect was less apparent. Although it is difficult to test in the field, the birds with higher survival rate should also experience greater longevity when extrinsic mortality is removed.
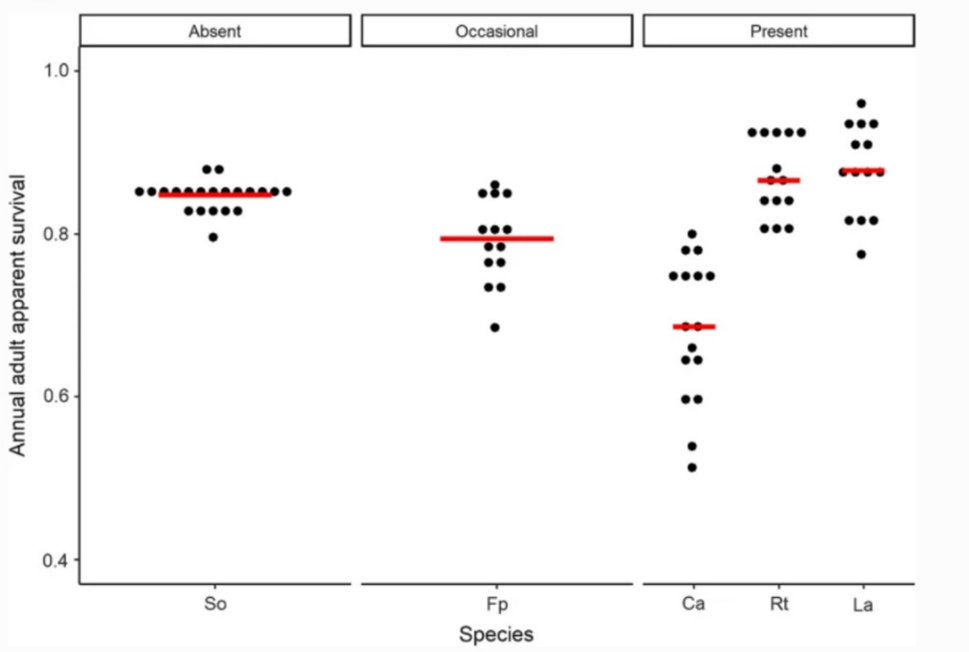
The social structure of a species may also be classified by their tolerance of members of their species; they may be open with their species, tolerant, or intolerant. One mammal with an extremely high longevity for its body mass is the naked mole rat, a 35-gram rodent with a remarkable maximum lifespan of an estimated 30 years (Edrey et al., 2011). Naked mole rats live a completely subterranean life, working in colonies of up to 300 individuals to support a singular breeding female (the queen). Unlike colonies of insects, the remarkable longevity of these rodents extends to the workers as well as the queen. However, the workers suffer higher rates of extrinsic mortality. Disputes in naked mole rat colonies are rare, except for females battling to become queen when a new one must be recruited. This cooperation allows the mole rats to function as an efficient team to maintain the colony. Other species are less friendly, and many have evolved aggression towards members of their own species driven by a variety of factors. Organisms may compete for food, territory, or mates, reducing the benefits of social interactions. Aggression leads to quicker senescence through the accelerated production and release of hormones that suppress the immune system, damage organs, and increase oxidative stress (Georgiev et al., 2013). More tolerant animals generally have greater longevity within a species, but there is no evidence of a concrete relationship between tolerance and longevity so far. One notable example of a long-lived solitary mammal is the South American tapir: one specimen named “George” lived to 39.6 years of age (Pinho, G. M., Gonçalves da Silva, A. et al, 2014).
Trends between longevity, organization levels and offspring membership
Mammals are a diverse group of creatures, and some are very family-oriented species that generally nurture their young during their first stages of life. Their social structure provides many different advantages depending on the familial dynamics and lifestyle (Foote, 2008).
When looking at the organizational level, we are referring to the possible social hierarchy within a certain group of animals from the same species; some species can be studied and considered as having no levels, one level or multiple levels of social organization. No organizational level mainly refers to mammals that travel alone, in groups or in families, without being a part of a larger whole (Prox & Farine, 2020). This is especially the case for territorial mammals, such as black bears and American red squirrels. One level considers mammals that travel in units that comprise a larger local community. An excellent example of this is gelada baboons; they travel in bands of varying sex ratios that collectively cooperate to share resources. Finally, a multilevel organization is the most complex form of social connection, where the community separates into squadrons that respectively contain smaller units. This is observable in mammals who work together and divide community tasks amongst units to fulfill unique duties, such as foraging, navigation and nurturing. This is seen in killer whales, whose communities are divided into core groups, bond groups and clan groups (Prox & Farine, 2020).
When it comes to longevity, the organizational level does provide great advantages with fluctuating magnitudes, both for the parents and their offspring; higher social complexity and order improve the efficiency in foraging, hunting, nurturing and notably resource management and distribution (Barocas et al., 2011). It also allows for better predatory defence, and stronger social bonds have been linked to improved health and longevity overall (Riehl & Strong, 2018). However, there are some speculated downsides that may arise from increased social complexity, including interspecific competition for resources, territorial disputes, and aggression amongst band members, which may have a considerable role in determining the well-being and longevity of a given species.
Whales are an excellent case study for this (Fig. 4). In the left-hand side graphs, lifespan is related to survivorship and fertility of different females from three whale species. While pilot whales aggregate in groups reaching up to thousands of individuals in a one-level social organization, where they roam the seas and feed off mainly squid, killer whales are predators hunting in smaller, more coordinated, and functional multi-level groups. We may notice that long-finned pilot whales live much longer than killer whales, while killer whales remain fertile longer. Variables like group size, resource scarcity, and even the studied populations (the data was extrapolated over decades of field studies, and dozens of different populations plot the graphs) play into this essential difference, but the general trend implies that females living in complex social structures benefit from optimal fecundity for a large majority of their lifetime, especially in the case of short-finned pilot whales (Foote, 2008).
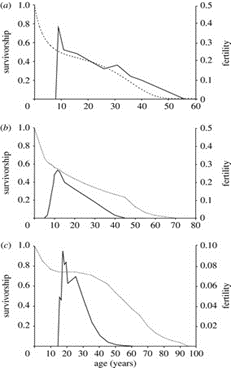
Another relatively familial and communal aspect to study about social structure within mammals is offspring membership: whether the offspring is nurtured by the parents or not. The duration of this care plays a significant role in the offspring’s longevity as well as the parents’. This characteristic of mammalian social dynamics can be classified in four main sections; absent, limited, extended or philopatric offspring membership to the community. Absent offspring attachments are rare, but, considering that almost 95% of mammalian species receive their nurturing from the mother, occur in all-male groups of, for example, baboons (Prox & Farine, 2020). Limited offspring attachment can be defined as around roughly a year or less of membership, such as with polar foxes whose cubs leave the den within their first winter. Eastern gray kangaroos are an excellent illustration of extended offspring membership, since the young remain under parental care even with generational overlap, although the once adult they tend to separate. Finally, philopatric offspring will typically remain with their parents and family for their entire life, possibly occasionally separating only to return in potential seasonal cycles, as it is with South American coatis (Prox & Farine, 2020).
When studying populations with different offspring memberships, the general trend implies that a longer attachment is favourable for the longevity of both the parent and the young. The offspring naturally receive better care for longer and thus develop with less predatory risk and better nutrients, which translates into an extended lifespan (Péron et al., 2019). Moreover, mothers who are more inclined to tend to the young counter the expenses of raising offspring by evolving through the ‘grandmother hypothesis,’ where not only do they gain parental experience to raise offspring more efficiently, they also promote longevity by providing function and purpose beyond sexual maturity and reproductive capability, which makes menopausal mammals live much longer past their fertility period to care for the next generation of children (Weladji et al., 2006). Figure 5 provides evidence for the trends in matrilineal mammalian communities, such as the semi-domestic reindeer (R. tarandus), the lion (P. leo) or the Sri lankan elephant (E. maximus maximus), and mammals in general.
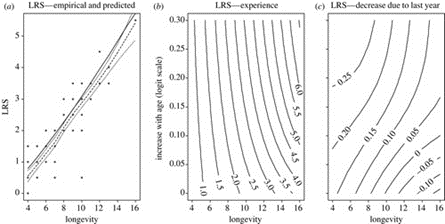
Greater social organization and hierarchy, along with more devoted parental care and offspring membership to their given community generally tend towards greater longevity for those individuals, given proper territorial and resource management.
Trends between longevity, sex ratio and group size
Many factors affect the longevity of a species and those can be extrinsic factors, such as predation or ability to forage efficiently, or intrinsic factors, such as health. In the case of mammals, where there is a wide array of different group sizes ranging from individual to groups of thousands of individuals, the idea of a greater group size leading to greater longevity due to the decrease in extrinsic risks of mortality, such as a lower risk of predation in smaller groups or “improved foraging efficiency and resource defence” (Lucas, 2020), has been put forth. Many studies have tried to see if this idea does exist in nature, however, the results are not clear. When there is an elevated mortality and lower average life expectancy, over time, species should evolve to quicken their pace of life and this “lowers selective pressure to remain fit and healthy” (Lucas, 2020) up to old age. In most cases, there is no clear relation between group size and longevity. In fact, in the case of artiodactyls, even toed ungulates such as pigs, goats, or cattle, a negative correlation has been shown, with larger groups leading to lower lifespans. Some have suggested that an increase in group size leading to greater longevity is only significant in “species that have already adopted a social mode of life” (Lucas, 2020) as they have evolved to rely greatly on the social structures present in their groups and would not survive as well without the given support of a group.
A different hypothesis is that there exists an optimal group size for each species that leads to maximum longevity. Although group living brings many benefits, it also has many drawbacks such as increased parasite infection. Therefore, sociality would only exist in species if the benefits they bring outweighs the costs. In the case of the social cliff swallow, “colony size is correlated with at least 13 different types of costs (e.g., parasitic infestation, brood parasitism) and at least 13 different types of benefits (e.g., predator-avoidance, information transfer)” (Gager et al., 2016). In many species, researchers have found that there exists an optimal group size leading to maximal longevity, with a steep drop in survival over a certain threshold. In socially foraging bats, group size seems to “optimize trade-off between foraging benefits from information transfer and acoustic confusion impairing prey detection performance” (Gager et al., 2016). Thus, for now, there is no clear trend between group size and longevity, most probably due to the sheer number of factors that influence sociality and longevity.
Another dimension in categorizing mammalian social structure would be the sex ratio in a group. Two significant categories are cooperative breeding species, where offspring receive care from members that are not their parents called helpers, such as in wolf packs, or polygynous species, which can be seen in gorillas, where one male mates with multiple females, while females mate only with one male at a time. Again, there are many factors that come into play with no clear trend being present. In some cases of cooperative breeders, helpers in queue to becoming breeders or in colonies that have limited breeding opportunities experience delayed reproduction which leads to greater longevity, as well as the dominant breeders, such as queens in colonies, who senesce more slowly than the other members of the group. There is also seen to be a sex-biased senescence in some species, such as in polygynous species. In order to compete successfully against other males, there is a trade between energetically costly traits that enhance reproductive success and other factors that increase survival, leading to reduced longevity in males (Greiner et al., 2014). A study done on the polygynous sac-winged bat S. Bilineata showed that males “increase in reproductive success until they reach the age of four” (Greiner et al., 2014), while females continue to produce offspring until they die (Fig. 6). In general, females have a more stable fitness component and reproductive success, while males vary greatly over time with a peak at age 4. Due to their lower reproductive success at older ages, males do not need to have as long a lifespan, leading to lower longevity. Females on the other hand are able to reproduce at older ages and therefore live longer than their male counterparts. Sex ratio can explain in part difference in longevity between sexes or roles in society although much more research can be done in this area.
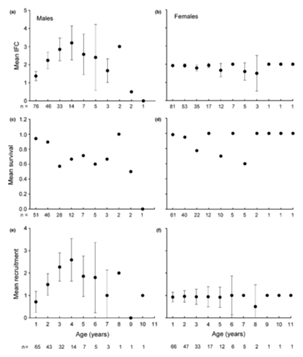
Trends between longevity, seasonal cycling and group stability
At first glance, seasonal variation in social organizations appears to be a binary characteristic. Either a species reshapes its social organization seasonally, or it does not. In actuality, seasonal variation is a relatively complex trait; it presents diverse adaptations in both the manner of variation, the ‘how and why,’ and the incidence of variation. By breaking seasonal variation down into these two traits, we can better speak to the relationship between seasonal variation and longevity, the ‘when.’
There are many manners in which seasonal variation in social organization can be expressed. Many species are known for “disbanding their groups to form pairs during the nonbreeding season (or vice-versa)” (Prox & Farine, 2020). Alternatively, some species, such as female sperm whales, are only non-solitary during the breeding season (Whitehead & Kahn, 1992). There are also species which have been found to have seasonal turnover between groups, such that the members that make up the group change significantly each season. A notable example of this is the female Asian elephant. This population experiences considerable seasonal turnover between groups whilst not noticeably impacting group size (Nandini et al., 2017). If the manner of variation provides the how and why, the incidence provides the when. Incidence is the metric for describing when this seasonal variation occurs. It often aligns with the seasons of the Gregorian calendar, with wet and dry seasons, or with the mating season of a species. The duration of periods of behavior is an important aspect to observe when measuring the impact of seasonal variations upon the longevity of an organism.
Generally, we can attribute seasonal variations in social organization to reproductive practices or to consequences of seasonal environmental changes such as the presence of predators and the availability of food. If a predator goes into hibernation for the season, their prey’s need for the protection afforded by a group is lessened. If food becomes scarce during the winter, herbivores might congregate in locations favorable to their sustainment. In early winter, clearings offer the best access to the favored food source of the Japanese sika deer, dwarf bamboo. As a result, sika deer have been observed to congregate in clearings in large groups during that time (Fig. 7). Essentially, this element of their seasonal cycling is environmentally imposed through the presence of their favored food being limited to certain locations during winter months. Further, the component of their seasonal variations preceding the winter is the breeding season which coincides with autumn. During the breeding season, the sika deer aggregate in larger groups than in the winter, with the objective of copulation leading to reproduction (Borkowski & Furubayashi, 1998).
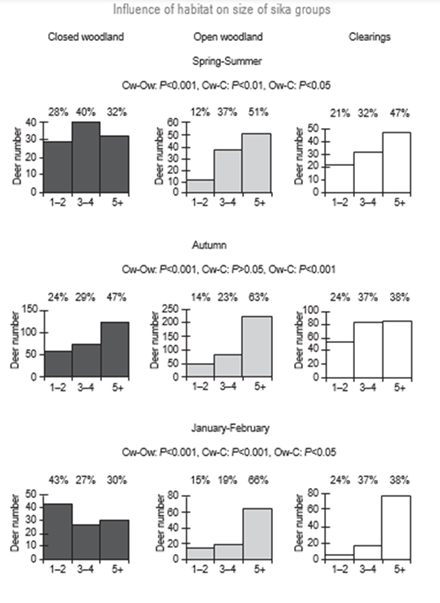
In both cases, we can attribute these variations in social organization to the objective of the continued survival of the species. Whilst this does not speak to the longevity of a species in the way its metabolism might, these trends display adaptations with the purposes of survival and reproduction. If sika deer did not group around abundant food sources in the winter, there would likely be a higher mortality rate due to starvation and thus a lower mean longevity of the species.
Earlier, we mentioned that the female Asian elephant has population turnover. Turnover in group membership is described as temporal stability. While some species are quite stable, others have high amounts of turnover. Thus, temporal stability can be broken down into four classes. Fluid stability, as seen in nyalas (Anderson, 1980), is typical in social organizations with a high degree of group changing. Cyclical stability, as seen in sika deer (Borkowski & Furubayashi, 1998), is seasonal turnover in membership. As such, a group may come together in the winter, but separate come spring. Long-lasting stability indicates few changes in a group over a lifetime. These changes can be provoked by demographic factors or resource availability (Prox & Farine, 2014). As seen in Thomas’ Langurs, if a group becomes too large or the usual sex ratio becomes unbalanced, individuals might depart the group (Sterck, 1997). Permanent stability indicates little to no changing of groups once adulthood has been reached (Prox & Faine, 2014).
Each of these forms of stability dictate other social and environmental factors that impact longevity of the species. If a different form of stability were attributed to a species, it could significantly impact longevity. If sika deer had fluid stability, they would not group in clearings during the winter. As a result, they would likely not be well fed during the winter and the rate of starvation would be higher. If Thomas’ Langurs abided by permanent stability as opposed to long-lasting stability, groups would become too large, leading to death by famine for lack of resources. Further, they might also experience such a massive gender imbalance that reproduction becomes sub-optimal. In both of these cases, the mean longevity of the species is negatively impacted.
Conclusion
The relationship between longevity of a species and its social organization is clearly of importance. The formation of primary units, tolerance, organization levels, offspring membership, sex ratio, group size, seasonal cycling and group stability all have distinct impacts upon the longevity of a species. If a couple of these characteristics were to be modified suddenly, it could wreak havoc upon a population. It could unbalance a population, increasing vulnerability to predators, decreasing access to natural resources, increasing intraspecies mortality or decrease the survival rates of the young. This could lead to extinction of a species. In effect, the social adaptations of a species are a barrier warding off threats of extinction and effectively padding its longevity. Thus, the intricate balance of adapted social characteristics found in each species is and will continue to be essential to longevity and survival.
References
Anderson, J. (1980). The social organisation and aspects of behaviour of the nyala Tragelaphus angasi Gray, 1849.
Barocas, A., Ilany, A., Koren, L., Kam, M., & Geffen, E. (2011). Variance in centrality within rock hyrax social networks predicts adult longevity. PLOS ONE, 6(7), e22375. https://doi.org/10.1371/journal.pone.0022375
Beauchamp, G. (2021). Flocking in birds increases annual adult survival in a global analysis. Oecologia, 197(2), 387-394. https://doi.org/10.1007/s00442-021-05023-5
Borkowski, J., & Furubayashi, K. (1998). Seasonal and diel variation in group size among Japanese sika deer in different habitats. Journal of Zoology, 245(1), 29-34. https://doi.org/10.1111/j.1469-7998.1998.tb00068.x
Edrey, Y. H., Park, T. J., Kang, H., Biney, A., & Buffenstein, R. (2011). Endocrine function and neurobiology of the longest-living rodent, the naked mole-rat. Exp Gerontol, 46(2-3), 116-123. https://doi.org/10.1016/j.exger.2010.09.005
Foote, A. D. (2008). Mortality rate acceleration and post-reproductive lifespan in matrilineal whale species. Biol Lett, 4(2), 189-191. https://doi.org/10.1098/rsbl.2008.0006
Gager, Y., Gimenez, O., O’Mara, M. T., & Dechmann, D. K. (2016). Group size, survival and surprisingly short lifespan in socially foraging bats. BMC Ecol, 16, 2. https://doi.org/10.1186/s12898-016-0056-1
Georgiev, A. V., Klimczuk, A. C., Traficonte, D. M., & Maestripieri, D. (2013). When violence pays: a cost-benefit analysis of aggressive behavior in animals and humans. Evol Psychol, 11(3), 678-699. https://www.ncbi.nlm.nih.gov/pubmed/23864299
Greiner, S., Nagy, M., Mayer, F., Knörnschild, M., Hofer, H., & Voigt, C. C. (2014). Sex-Biased Senescence in a Polygynous Bat Species. Ethology, 120(2), 197-205. https://doi.org/10.1111/eth.12193
Kamilar, J. M., Bribiescas, R. G., & Bradley, B. J. (2010). Is group size related to longevity in mammals? Biol Lett, 6(6), 736-739. https://doi.org/10.1098/rsbl.2010.0348
Lucas, E. R., & Keller, L. (2020). The co‐evolution of longevity and social life. Functional Ecology, 34(1), 76-87. https://doi.org/10.1111/1365-2435.13445
Moller, A. P. (2006). Sociality, age at first reproduction and senescence: comparative analyses of birds. J Evol Biol, 19(3), 682-689. https://doi.org/10.1111/j.1420-9101.2005.01065.x
Nandini, S., Keerthipriya, P., & Vidya, T. N. C. (2017). Seasonal variation in female Asian elephant social structure in Nagarahole-Bandipur, southern India. Animal Behaviour, 134, 135-145. https://doi.org/https://doi.org/10.1016/j.anbehav.2017.10.012
Péron, G., Bonenfant, C., Lemaitre, J.-F., Ronget, V., Tidiere, M., & Gaillard, J.-M. (2019). Does grandparental care select for a longer lifespan in non-human mammals? Biological Journal of the Linnean Society. https://doi.org/10.1093/biolinnean/blz078
Prox, L., & Farine, D. (2020). A framework for conceptualizing dimensions of social organization in mammals. Ecol Evol, 10(2), 791-807. https://doi.org/10.1002/ece3.5936
Riehl, C., & Strong, M. J. (2018). Stable social relationships between unrelated females increase individual fitness in a cooperative bird. Proceedings. Biological sciences, 285(1876). https://doi.org/10.1098/rspb.2018.0130
Sterck, E. H. (1997). Determinants of female dispersal in Thomas langurs. American Journal of Primatology, 42(3), 179-198.
Toth, A. L., Sumner, S., & Jeanne, R. L. (2016). Patterns of longevity across a sociality gradient in vespid wasps. Curr Opin Insect Sci, 16, 28-35. https://doi.org/10.1016/j.cois.2016.05.006
Weladji, R. B., Gaillard, J. M., Yoccoz, N. G., Holand, O., Mysterud, A., Loison, A., Nieminen, M., & Stenseth, N. C. (2006). Good reindeer mothers live longer and become better in raising offspring. Proceedings. Biological sciences, 273(1591), 1239-1244. https://doi.org/10.1098/rspb.2005.3393
Whitehead, H., & Kahn, B. (1992). Temporal and geographic variation in the social structure of female sperm whales. Canadian Journal of Zoology, 70(11), 2145-2149. https://doi.org/10.1139/z92-289