Abstract
Communication in the animal kingdom has been explored by humans for centuries. As the species with the most elaborate form of communication, language, we seek to understand how other species interact amongst themselves. Though we are unable to comprehend their communication in the way that we understand our own speech, we are still able to observe different forms of communication and evaluate how they occur in a physical manner. This paper is a portfolio of four different categories of communication – visual, tactile, auditory, and electric. In each category, one to two different animal’s communication techniques are explored with a focus on the physics of it. Visual communication is explored with structural colour of bird feathers and circularly polarized light in mantis shrimp; tactile communication is investigated using the analysis of a horses’ kick; auditory communication is inspected by looking at echolocation and social calls in bats; and electrocommunication is examined by studying electric signals in the brown ghost knifefish. Though this is only a taste of the vast variety of communication in the animal kingdom, it is already quite humbling to see that while we may have language, we are far from having the most unique form of communication.
Introduction
Communication is defined as an exchange of information. In the animal kingdom, these exchanges occur through visual, tactile, auditory, and electric modes. To better understand each of these communication methods, one to two poignant examples will be discussed. As we shall see, birds and mantis shrimp both make extensive use of visual communication, albeit in entirely different ways, while bats are masters of acoustic communication, and horses utilize tactile “kicks” to express emotion and dominance. Finally, we will end by exploring electrocommunication in weakly electric fish. In each of these examples, a lack of verbal correspondence by no means signifies a lack of complexity. On the contrary, complex and nuanced communication is observed in all modalities discussed.
Visual Communication
Earth gets light from the sun, and over the 3.5 billion years of life on this planet (National Museum of Natural History, n.d.) organisms have evolved to utilize this light. While the use of sunlight may have originally been for photosynthesis, it is now used by most species as a way to observe the world around them. When evolution gave animals eyes, it not only gave them a way to evade predators and find prey, but it also gave them a way to receive visual stimuli and thus to communicate.
Birds — Structural Colour
Birds are a prime example of visual communication in the animal kingdom. The incredible array of vibrant bird plumage – seen in peacocks to rainbow lorikeets – plays a role in social behaviour, courtship, parent-offspring relationship, and interspecific recognition (Price-Waldman & Stoddard, 2021).
Some plumage colours are produced using pigments like melanins and carotenoids (Price-Waldman & Stoddard, 2021), however, iridescent as well as some non-iridescent colours (like blue and green) are made using a technique called structural colouration.
Bird feathers are composed of a quill, barbs, and barbules (Fig. 1). Depending on the bird species the structural colouration can stem from either the barbs or the barbules (Noh et al., 2010; Yin et al., 2012). Regardless of where the structural colour originates, the fundamental technique behind the colour is the same. Within the barbs/barbules there are nanostructures made of spongy keratin that form photonic structures (Noh et al., 2010; Prum et al., 2009) that reflect or scatter light to cause the constructive interference of certain frequencies (McGuire et al., n.d.), while the rest interferes destructively. In the case of a blue feather, blue wavelengths constructively interfere while the wavelengths of other colours destructively interfere.
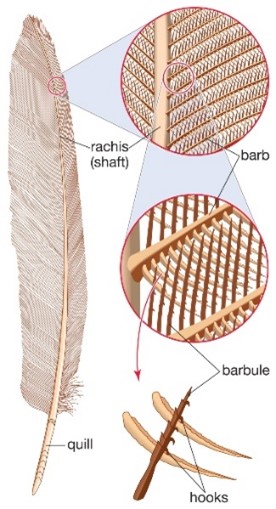
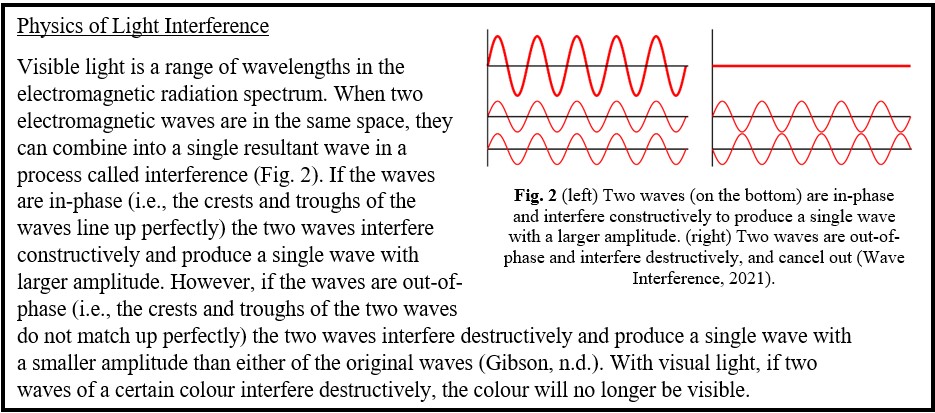
For non-iridescent colours (like solid blue or green), the colour of the feather appears the same no matter the angle from which the feather is observed. These feathers have nanostructures that are disordered (Ball, 2012; McGuire et al., n.d.), which scatter the light in a diffuse manner so that uniform colouration is observed from all angles (Ball, 2012). These structures are called photonic glasses (McGuire et al., n.d.). In iridescent colours, the feather colour changes as it is viewed from different angles. These feathers have nanostructures that are ordered and are called photonic crystals (McGuire et al., n.d.).
Mantis Shrimp — Circularly Polarized Light
In contrast to the vibrant visual displays of birds are organisms that communicate visually in ways that are so unique that no other species can see it. Mantis shrimp (Fig. 3) are a group comprised of over 350 species (The Editors of Encyclopaedia Britannica, 2016) that have a unique ability to see and produce circularly polarized light (Gagnon et al., 2015).
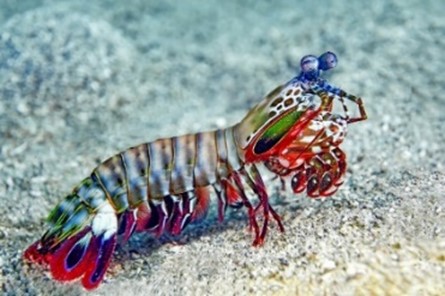
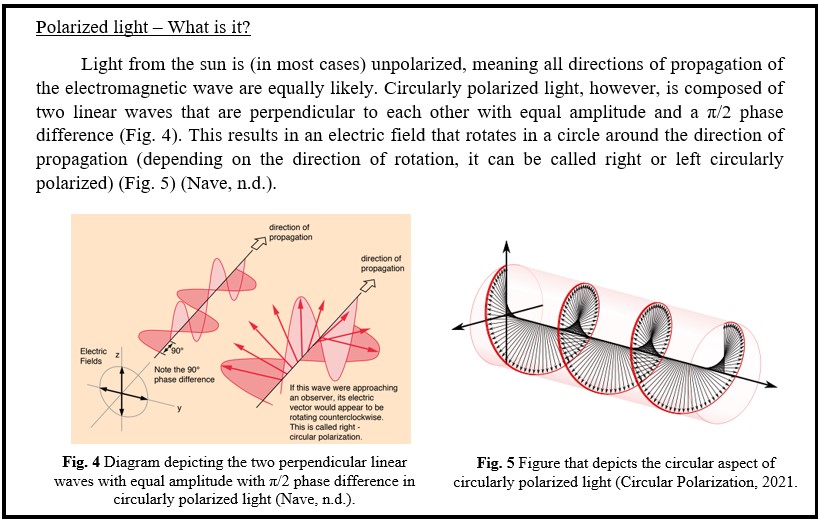
Though it has been known for some time that mantis shrimp could see and produce circularly polarized light, it is only in the last decade that there has been evidence that they use this type of light to communicate amongst themselves (Gagnon et al., 2015).
Mantis shrimp are strong: they have the quickest punch of any known animal capable of breaking aquarium strength glass (Yong, 2015). They are also territorial, and if one were to wander into a burrow already occupied by another mantis shrimp, a fight could break out and lead to serious injury or death (Yong, 2015). However, it appears that mantis shrimp use circularly polarized light to indicate their presence to conspecifics. Once a mantis shrimp senses the presence of another in a burrow, they avoid it to prevent conflict. This discovery was made in a study by Gagnon et al. (2015) where mantis shrimps were placed in tanks with two burrows, one with unpolarized light, and the other with circularly polarized light. It was found that shrimp were more likely to pick the burrow without the circularly polarized light. This indicated that circularly polarized light was used by the mantis shrimp to communicate their presence within the species to avoid conflict (Gagnon et al., 2015).
Tactile Communication
From social bonding to assertion of dominance, from mating to infant care, animals interact through physical behaviour and touch. This is known as tactile communication, a form of communication with physical interactions and no linguistics commonly observed in social situations between animals. The type of touch that is delivered can convey a specific and unique message.
Horses use kicking as a powerful form of communication. A horse’s kick can occur both ungoverned and controlled. To express enjoyment, a horse can be observed prancing in its environment, with occasional small kicks between the vertical intervals. This smaller force kick occurs heedlessly due to stimulus of playfulness (Kelly et al., 2021). To assert dominance over another horse, an alpha horse will threaten with pinned ears, and aim their backside towards its target, before unleashing a forceful kick to the beta horse. This delivery of kicks is a social behaviour called “Herd Hierarchy,” used to establish an order of dominance (Fureix et al., 2012).
In a social context, with the combination of the magnitude of the force and the angle at which a horse’s kick is delivered, a horse can convey emotion. Analysis of a distinct “horse kick” can be done through force measurements (Fig. 6).
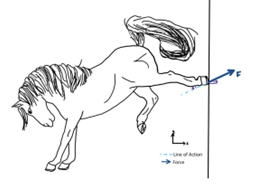
Measurements can be taken to approximate the magnitude of the force of a horse’s kick by assessing the linear interrelation between an acting force and an induced elastic deformation (∆ℓ) using a force meter. The force value (refer to Equation 1), assessed by the force meter, is the horizontal component of a force (Benthien et al., 2020).
F = ME \cdot \Delta l \space \space \space \space \space \space (Equation \space 1)
Where ME = modulus of elasticity (N mm2) (a constant of the force meter spring) and Δl = deformation of spring (mm).
Knowing the horizontal component, to find the magnitude of the kick force, use Equation 2.
F = F_{x} \cdot sec \theta \space \space \space \space \space \space (Equation \space 2)
Where Fx = force calculated by Equation 1 and θ = angle between force and x-axis (horzontal axis).
Through quantification of the mechanical force of a horse’s kick, it can be recognized that the magnitude of force of a horse’s kick can vary. Depending on the social context, this form of tactile communication, the “kick,” conveys different messages and emotions.
Auditory Communication
A dog barking, a cat meowing and a bee buzzing are all forms of auditory communication. Auditory communication occurs when a species produces sound to communicate with its environment. Different animal species make unique sounds and can therefore be identified by their form of auditory communication. For example, if one hears a bark, intuitively, a dog is presumed to be close by. To further understand how animals communicate vocally it is useful to understand the physical concept upon which auditory communication is based: sound waves.
Sound Waves
Sound waves (longitudinal waves) cause vibrations of air molecules which results in areas of high and low pressure. For this reason, sound waves are also referred to as pressure waves and can be expressed using the following formula (Halliday et al., 2014):
\Delta P = \Delta P_{max} sin(kx \pm wt \pm \phi) \space \space \space \space \space \space (Equation \space 3)
Where ΔP = change in pressure (Pa), ΔPmax = maximum change in pressure (Pa), k = 2π / λ = wave number (1/m), w = 2π / T = 2πf = angular frequency (rad/s), and Φ = initial phase (rad).
The waves are transmitted outwards from the point of origin in a radial fashion. Waves can have different frequencies, wavelengths, velocities, periods, and amplitudes. The wavelength and frequency are inversely proportional to one another, which means that lower frequency sound waves can travel longer distances compared to higher frequency sounds. When a sound wave hits an object, it can be reflected, refracted, diffracted, or transmitted. For the purpose of this paper, only reflected sound waves will be discussed. Reflection occurs when a sound wave reaches a hard medium and undergoes a change in direction thereby reflecting the wave back to the emitter with the same frequency (Halliday et al., 2014). Bats have evolved to take advantage of the properties of sound waves. Bats, part of the chordate phylum, have a clever way of communicating vocally that relies on the physical properties of sound waves. Echolocation and social calls will further be explored as they constitute the main forms of bat communication.
Echolocation
Bats use echolocation for a variety of functions, including navigation, hunting, and, most relevant to this paper, communication with other bat species. When bats echolocate they produce sound waves by projecting air through their vocal chords at frequencies higher than the human ear is capable of hearing (G. Jones & Holderied, 2007). The sounds they produce are rather simple and short (often only one syllable) because high frequency sounds waves entail proximity of low and high-pressure areas (eq. 3). Consequently, the medium in which the wave travels is compressed and causes the sound to attenuate more rapidly. The sound waves travel through air and are reflected once they encounter an object, a phenomenon known as an echo (Fig. 7). Once the sound wave returns to the bat, the waves enter the ear canal and navigate towards the eardrum where vibrations occur. Further processing within the brain allows interpretation of the sound wave.
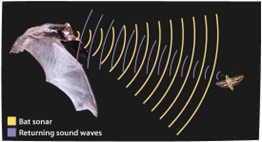
Fig. 7 Visual representation of emitted and echoed sound waves (echolocation) (Hagen, 2009).
Echolocation can provide an exclusive communication channel to bats because unlike most animals, they can produce and hear sound between 9 and 200 kHz (Heffner et al., 2019). Sounds produced during echolocation have high frequencies and are thus audible to very few species other than bats.
Bats that echolocate in groups may face challenges when conspecifics emit echolocation calls of similar frequencies. This causes acoustic interference between the desired echoes and the sound waves produced by other echolocating bats, a phenomenon known as jamming. To avoid jamming, bats change their frequencies as to emit calls that differ from their conspecifics. Such behaviour is known as the jamming avoidance response (JAR) (T. K. Jones & Conner, 2019).
Echolocation can provide information on age, sex and proximity through processing of echoes and calls from conspecifics; however, bat communication often takes place through social communication calls (Wright et al., 2013).
Social Communication Calls
The most commonly used method of communication for bats is social communication calls. These calls consist of low frequency and long wavelength sound waves, capable of carrying sound long distances. The calls may consist of complex messages of several syllables (Kanwal & Rauschecker, 2007). Bats produce several types of calls including calls of distress, agony, infant isolation, territory, and mating songs.
Infant isolation calls are a form of communication between a mother and her offspring. The sound emitted by the infants are frequency-modulated (FM) waves, meaning that the frequency of the wave varies, allowing for transmission of more complex information. Mothers can identify and locate their offspring through recognition of their call. Although bats belonging to the same social group produce similar isolation calls, mothers have developed mechanisms which allow them to successfully identify their own child. It is believed that female bats store a signal template in their memory and can compare it to incoming infant isolation signals to properly identify their own offspring (Bohn et al., 2007).
Male bats are known for singing, especially during the mating period, as it serves to attract females. Just as some animals attract mates with their bright and beautiful feathers, bats attract females with their capability of creating charming songs. Multisyllabic and structured territorial songs are a subcategory of mating songs. They are used to resolve territorial disputes between male bats and may also attract nearby females. The typical structure of a territorial song starts with a few seconds of constant frequency syllables, followed by a frequency modulated part (Knörnschild et al., 2017). Songs represent the most complex form of communication between bats, and are made up of chirps, composed of two distinct syllable types, trills, and buzzes (Bohn et al., 2008). The songs are delivered at rather low frequencies, audible to the human ear, and last approximately 1.6 seconds. Territorial songs repel bats from other social groups, and inform females of group size, which may be of importance if one is looking to mate. Females gravitate towards familiar songs delivered by bigger group sizes, as a larger group entails more mating options for the female to choose from.
Bats have proven to be heavily dependent on auditory communication; namely, echolocation and social communication calls. Their mode of communication relies on properties of sound waves and internal processing of signals within the bat ear.
Electrocommunication
Electrocommunication and Electrolocation
Electrocommunication is a form of communication used by around 350 species of weakly electric fish inhabiting the neotropical freshwater systems of South America and Africa. Weakly electric fish are capable of both detecting electric fields and generating their own (i.e., they are electroreceptive and electrogenic) using a specialized electric organ (Nelson, 2011). Unlike strongly electric fish, the most well-known of which is the electric eel (Electrophorus electricus), the signals emitted by weakly electric fish rarely exceed 1 V/cm in strength (Nelson, 2011; Stoddard, 2009).
Weakly electric fish generate electric fields primarily for the purpose of electrolocation, i.e., they use their electric fields to image their surroundings. This ability arises because objects that are more or less resistive than water will respectively cause a local intensification or diminution of the fishes’ electric fields. Capacitive objects, including many living organisms, will also distort the fish’s electric waveform. These minute changes are detected by cutaneous ampullary electroreceptors and interpreted to allow weakly electric fish to ‘see’ at night or in perpetually turbid waters (Stoddard, 2009).
Although weakly electric fish are constantly discharging electric signals for the purpose of electrolocation, they can modulate their signals in social situations to convey a variety of messages. This is the basis of electrocommunication. Frequency is the most common parameter that is varied, however changes in amplitude and waveform may also be significant. In some cases, the unmodified electric organ discharge (EOD) is in itself meaningful as different species, sexes and individuals exhibit unique waveforms that can be recognized by other electroreceptive fish (Stoddard, 2009).
Electrocommunication in the Brown Ghost Knife-fish (Apteronotus Leptorhynchus)
For the rest of this section, electrocommunication in the brown ghost knifefish will be discussed (Fig. 8). This nocturnal species lives in extremely high densities in the wild and so they make extensive use of electric signals to maintain social order and minimize fighting (Buehler, 2021). The role of EODf in signalling dominance in this species will be described, followed by an outline of the significance of various frequency modulations (JARs, chirps, rises, yodels and dips) in electrocommunication (Zakon et al., 2002).
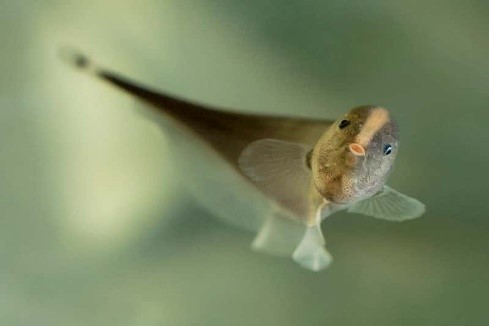
Sexual Dimorphism in Frequency and Dominance
In brown ghost knife-fish, EOD frequency (EODf) and dominance are positively correlated. Alternately stated, higher-frequency individuals are more dominant as compared to lower-frequency conspecifics and males are dominant over females, who discharge in a lower frequency range than males (Zakon et al., 2002).
The ‘frequency hierarchy’ among males can be observed in agonistic situations. If two males are placed in a tank with a single shelter, the higher-frequency individual is more likely to successfully claim the refuge and this individual will chirp more frequently at the subordinated male. In addition, if several males and females are placed together in a tank, the highest-frequency male will be the only one to spawn with each of the females (Zakon et al., 2002).
It is not clear whether an analogous hierarchy is established among females, however females that are unable to compete for shelters often have lower EOD frequencies than those who manage to secure shelter (Zakon et al., 2002).
The Jamming Avoidance Response
When two brown ghosts with frequencies within ± 2-10 Hz come into close proximity, their EOD waveforms will interfere with each other or ‘jam’, making electrolocation and electrocommunication near impossible for both individuals. During nocturnal hours, brown ghosts avoid this problem by swimming away from conspecifics with similar EOD frequencies however, during the day this is not possible as multiple fish must often share a limited number of dark shelters. Hence, to minimize jamming the brown ghost with the higher frequency reflexively increases its EODf. This behavior is called the Jamming Avoidance Response (JAR) (Tallarovic & Zakon, 2005; Zakon et al., 2002).
The significance of the JAR in electrocommunication is that brown ghosts choose whether to perform this reflex or not. In aggressive encounters, brown ghosts intentionally jam rivals even though this impairs their own electrolocation abilities. Interestingly, brown ghosts will even try to jam artificial electric signals (Fig. 9). Hence, intentional jamming communicates threat in this species (Tallarovic & Zakon, 2005).
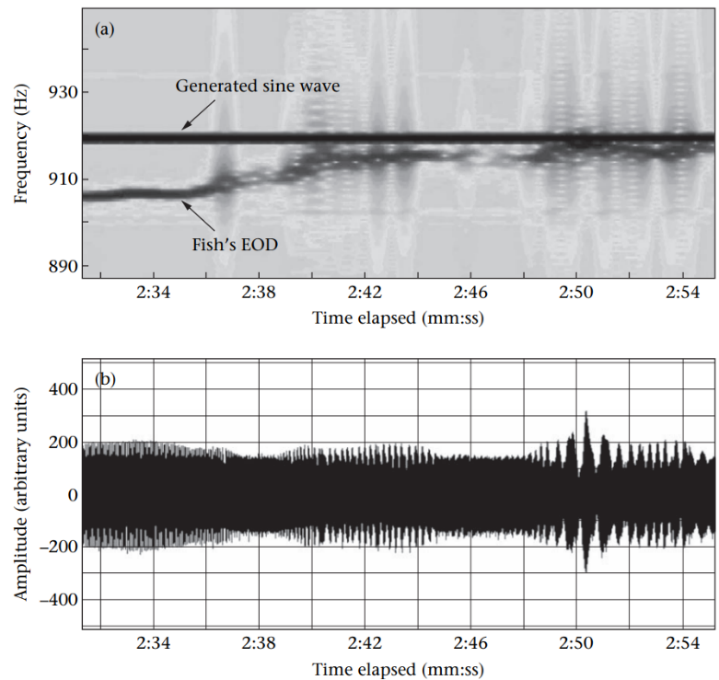
Chirps
Chirps are characteristically generated by males, although females are also capable of chirping. There are four types of chirps (types 1, 2, 3 and 4) that differ in duration and frequency excursion. Type 1 and 2 chirps are short (on the order of 20 ms) and they are used to communicate aggression in agonistic situations. By contrast, type 3 and 4 chirps can last for hundreds of milliseconds, and they are reserved for mating. Before releasing an egg, female brown ghosts emit a volley of rapid chirps (3-16 ms) that is followed by a long-duration chirp once the egg is laid. These signals are almost certainly designed to alert the male and thereby coordinate the mating process (Zakon et al., 2002).
Rises
Rises are gradual increases in EODf that can last up to 10 s. Rises have traditionally been interpreted by scientists as submissive cues because juvenile males make rises more often than dominant, mature males in response to an artificial playback signal. Nonetheless, it is more likely that function as provocative signals. In experiments in which a pair of fish were forced to compete for a refuge, the ‘loser’ made multiple rises that triggered aggressive attacks from the ‘winner’. Individuals that made these rises were more likely to secure shelter in future aggressive encounters, suggesting that rises enable subordinate knifefish to climb the social hierarchy (Zakon et al., 2002).
Yodels and Dips
Yodels and dips are the least-understood electric signals produced by brown ghosts. A yodel consists of a rapid spike in EODf and an exponential decline to baseline. Since yodeling results in a temporary increase in EODf, it could serve to convey a false air of dominance. Indeed, females competing of a shelter have been known to yodel. A dip, however, is a rare decrease in EODf by 10-20 Hz over 10-100 ms. The meaning of dips and the mechanism by which they are produced is unknown (Zakon et al., 2002).
Discussion and Conclusion
As has been expounded in this essay, animal communication is by no means limited to the verbal exchange of information. Broadly speaking, animals communicate using visual, tactile, auditory, and even electrical cues. Although the mode of communication among animals varies, a discussion of the communication methods of a few specific species revealed some interesting parallels. For example, mantis shrimp, horses, and electric fish are all concerned with signaling dominance while birds, bats, and electric fish have evolved specialized forms of communication for the purpose of courtship. Another fascinating commonality that was explored is the fact that animals make abundant use of physical laws – whether acoustic, mechanical, optical, or electrical – to convey meaning. This great diversity and sophistication places human communication in a wider context and causes us to question what communication truly represents on a philosophical level.
References
Ball, P. (2012). Nature’s Color Tricks. Scientific American, 306, 74-79. https://doi.org/10.1038/scientificamerican0512-74
Benthien, J. T., Ohlmeyer, M., Gäckler, S., Krause, A., & Al Samarraie, A. (2020). Experimental determination of the impact energy of horse kicks. Biosystems Engineering, 195, 54-63. https://doi.org/https://doi.org/10.1016/j.biosystemseng.2020.04.009
Bohn, K. M., Schmidt-French, B., Ma, S. T., & Pollak, G. D. (2008). Syllable acoustics, temporal patterns, and call composition vary with behavioral context in Mexican free-tailed bats. The Journal of the Acoustical Society of America, 124(3), 1838-1848. https://doi.org/10.1121/1.2953314
Bohn, K. M., Wilkinson, G. S., & Moss, C. F. (2007). Discrimination of infant isolation calls by female greater spear-nosed bats, Phyllostomus hastatus. Animal Behaviour, 73(3), 423-432. https://doi.org/https://doi.org/10.1016/j.anbehav.2006.09.003
Buehler, J. (2021, February 17). Knifefish use electric fields to develop a complex social hierarchy. NewScientist. Retrieved October 8, 2021, from https://www.newscientist.com/article/2268008-knifefish-use-electric-fields-to-develop-a-complex-social-hierarchy/
Circular polarization. (2021, August 25). In Wikipedia. Retrieved October 8, 2021, from https://en.wikipedia.org/w/index.php?title=Circular_polarization&oldid=1040527783
Fureix, C., Bourjade, M., Henry, S., Sankey, C., & Hausberger, M. (2012). Exploring aggression regulation in managed groups of horses Equus caballus. Applied Animal Behaviour Science, 138(3-4), 216-228. https://doi.org/10.1016/j.applanim.2012.02.009
Gagnon, Y. L., Templin, R. M., How, M. J., & Marshall, N. J. (2015). Circularly Polarized Light as a Communication Signal in Mantis Shrimps. Current Biology, 25(23), 3074-3078. https://doi.org/10.1016/j.cub.2015.10.047
Gibson, G. N. (n.d.). 5.2 Constructive and Destructive Interference. University of Connecticut. Retrieved October 8, 2021, from https://www.phys.uconn.edu/~gibson/Notes/Section5_2/Sec5_2.htm
Hagen, E. (2009, November 4). Echolocation. ASU – Ask A Biologist. Retrieved October 8, 2021, from https://askabiologist.asu.edu/echolocation
Halliday, D., Resnick, R., & Walker, J. (2014). Fundamentals of Physics, Extended (10 ed.). Wiley.
Heffner, R. S., Koay, G., & Heffner, H. E. (2019). Bats are unusually insensitive to brief low-frequency tones. Journal of Comparative Physiology A, 205(4), 583-594. https://doi.org/10.1007/s00359-019-01349-x
Jones, G., & Holderied, M. W. (2007). Bat echolocation calls: adaptation and convergent evolution. Proceedings of the Royal Society B: Biological Sciences, 274(1612), 905-912. https://doi.org/10.1098/rspb.2006.0200
Jones, T. K., & Conner, W. E. (2019). The jamming avoidance response in echolocating bats. Communicative & Integrative Biology, 12(1), 10-13. https://doi.org/10.1080/19420889.2019.1568818
Kanwal, J. S. & Rauschecker, J. P. (2007). Auditory cortex of bats and primates: managing species-specific calls for social communication. Frontiers in Bioscience, 12(8-12), 4621. https://doi.org/10.2741/2413
Kelly, K. J., McDuffee, L. A., & Mears, K. (2021). The Effect of Human–Horse Interactions on Equine Behaviour, Physiology, and Welfare: A Scoping Review. Animals, 11(10), 2782. https://doi.org/10.3390/ani11102782
Knörnschild, M., Blüml, S., Steidl, P., Eckenweber, M., & Nagy, M. (2017). Bat songs as acoustic beacons – male territorial songs attract dispersing females. Scientific Reports, 7(1). https://doi.org/10.1038/s41598-017-14434-5
McGuire, J., Neophytou, A., & von Raesfeld, A. (n.d.). Structural Color. Manoharan Lab at Harvard University. Retrieved October 8, 2021, from https://manoharan.seas.harvard.edu/structural-color
National Museum of Natural History. (n.d.). Early Life on Earth – Animal Origins. Smithsonian. Retrieved October 8, 2021, from https://naturalhistory.si.edu/education/teaching-resources/life-science/early-life-earth-animal-origins
Nave, C. R. (n.d.). Classification of Polarization. HyperPhysics. Retrieved October 8, 2021, from http://hyperphysics.phy-astr.gsu.edu/hbase/phyopt/polclas.html
Nelson, M. E. (2011). Electric fish. Current Biology, 21(14), R528-R529. https://doi.org/10.1016/j.cub.2011.03.045
Noh, H., Liew, S. F., Saranathan, V., Prum, R. O., Mochrie, S. G. J., Dufresne, E. R., & Cao, H. (2010). Double scattering of light from Biophotonic Nanostructures with short-range order. Optics Express, 18(11), 11942. https://doi.org/10.1364/oe.18.011942
Price-Waldman, R., & Stoddard, M. C. (2021). Avian Coloration Genetics: Recent Advances and Emerging Questions. Journal of Heredity, 112(5), 395-416. https://doi.org/10.1093/jhered/esab015
Prum, R. O., Dufresne, E. R., Quinn, T., & Waters, K. (2009). Development of colour-producing β-keratin nanostructures in avian feather barbs. Journal of The Royal Society Interface, 6(suppl_2), S253-S265. https://doi.org/10.1098/rsif.2008.0466.focus
Stoddard, P. K. (2009). Electric Signals & Electric Fish Philip Stoddard’s lab at FIU. Retrieved October 8, 2021, from https://efish.fiu.edu/publications/Stoddard_Electric_Signals_2009.pdf
Tallarovic, S. K., & Zakon, H. H. (2005). Electric organ discharge frequency jamming during social interactions in brown ghost knifefish, Apteronotus leptorhynchus. Animal Behaviour, 70(6), 1355-1365. https://doi.org/https://doi.org/10.1016/j.anbehav.2005.03.020
The Editors of Encyclopaedia Britannica. (2016, April 18). Mantis Shrimp. Encyclopaedia Britannica. Retrieved October 8, 2021, from https://www.britannica.com/animal/mantis-shrimp
The Editors of Encyclopaedia Britannica. (2019, January 30). Feather. Encyclopedia Britannica. Retrieved October 8, 2021, from https://www.britannica.com/science/feather
University of California, Irvine. (2020, August 17). Mantis shrimp’s dactyl clubs could hold secrets to more resilient surfaces for human use. Science X. Retrieved October 8, 2021, from https://phys.org/news/2020-08-mantis-shrimp-dactyl-clubs-secrets.html
Wave interference. (2021, October 4). In Wikipedia. Retrieved October 8, 2021, from https://en.wikipedia.org/w/index.php?title=Wave_interference&oldid=1048150235
Wright, G., Chiu, C., Xian, W., Moss, C., & Wilkinson, G. (2013). Social calls of flying big brown bats (Eptesicus fuscus) [Original Research]. Frontiers in Physiology, 4. https://doi.org/10.3389/fphys.2013.00214
Yin, H., Dong, B., Liu, X., Zhan, T., Shi, L., Zi, J., & Yablonovitch, E. (2012). Amorphous diamond-structured photonic crystal in the feather barbs of the scarlet macaw. Proceedings of the National Academy of Sciences, 109(27), 10798-10801. https://doi.org/doi:10.1073/pnas.1204383109
Yong, E. (2015, September 23). Mantis Shrimps Avoid Deadly Fights by Pummeling Each Other. The Atlantic. https://www.theatlantic.com/science/archive/2015/09/mantis-shrimps-fights-beating-each-other-up/406606/
Zakon, H., Oestreich, J., Tallarovic, S., & Triefenbach, F. (2002). EOD modulations of brown ghost electric fish: JARs, chirps, rises, and dips. Journal of Physiology-Paris, 96(5), 451-458. https://doi.org/https://doi.org/10.1016/S0928-4257(03)00012-3