Abstract
From the first living beings (bacteria, protozoa) to that of the most organized beings (mammals, insects, etc.), chemical communication, in the form of molecular exchange, has been an essential and universally used communication system. However, this system has only been studied for less than a hundred years, while the vision or hearing systems have been known, in broad outlines, for several centuries. Originally, most researchers focused on chemical communication between insects. Therefore, in this overview, we want to show the critical role of chemical communication in mutualism that involves insects. We discover a surprising mutualistic relationship between butterfly larvae and ants: firstly, to control the ants, the larvae must secrete nectar, a sweetened liquid. Once this step is done, the relationship will start. Chemical communication can also help avoid conflict between bees and ants, promoting a fair interaction between these species. By leaving a specific odor, the ants send a chemical signal to the honeybees to avoid flowers that they, the ants, have visited. Furthermore, we find out that some species employ chemical communication for all activities in their life. Specifically, we will talk about parasitoid wasps that can benefit most from chemical communication; theses hymenopteran superfamilies rely on this method for host detection, reproduction, and mutualistic relationship with plants. Finally, we discover that there are still many works to fully understand the role of chemical communication in species relationships. That is the case for Drosophila-yeast interaction, which has not proven to be mutualistic and to fully depend on chemical communication. The number of experiments is still limited and shows that the yeast preference varies from one genotype to another.
Introduction
The natural world constitutes an immense number of species coexisting in sophisticated ecological systems, seemingly without a means of communication. Despite the diversity of species and interspecies interactions, chemical communication effectively functions as a universal means of communication. Symbiotic and parasitic relationships between very different species exist due to chemical communication, whether it is between insects, wasps and plants, or fungi and flies. These relationships are common amongst insects because they reside in busy and highly competitive environments, where their offspring are untended for. The species have thus adopted means to communicate with each other, maximizing their and their offspring’ chances of survival. This paper examines the relationships of the nectar-protection transaction between butterfly larvae and ants, chemical identification that reduces competition between bees and ants, the numerous interspecies interactions of the parasitoid wasps through chemical detection, and how immotile yeasts move by chemically signally to flies to help them.
Butterfly Larvae Reward-Protection Relationship with Ants
Insects are among the least protected species in the animal kingdom when it comes to parental care. Their larvae have thus adapted mechanisms to protect themselves from parasitic and or predatory species. One such family of insects, the lycaenidae, consisting of butterflies, form a relationship with different species of ants as protection. The lycaenid larvae produce a nutritious secretion in exchange for protection and care from neighbouring ant species.
Around a third of species in lycaenidae are observed to associate in some way with ants, whether through facultative — temporary and non-essential — or obligate associations (Pierce & Elgar, 1985). This mechanism has given rise to the development of organs for maintaining such a relationship, namely pore cupola organs (PCOs), and dorsal nectary organs (DNOs) (Pierce et al., 2002). The secretions from these organs help the caterpillar communicate and coexist peacefully among ants.
The pore cupola organs excrete substances that help pacify the surrounding ants (Pierce et al., 2002). While ants are known to be socially aggressive, particularly towards caterpillars, they are found to ignore or interact peacefully with lycaenid caterpillars (Daniels et al., 2004). The substance produced is suggested to mimic the pheromones of the ant brood, communicating to and manipulating the ants into taking care of the larvae (Pierce et al., 2002). Ants are observed to lick frequently and touch the larvae with their antennae, and sometimes even bring them into their nest, where they are deposited in the brood chambers (Pierce et al., 2002). This chemical mimicry is exploited by the parasitic species of lycaenids, Maculinea rebeli, who, after being deposited in the brood chamber, eat the brood (Akino et al., 1999). However, this is not the case for all species, and the way the larvae pacify the ants chemically is still unclear.
The dorsal nectary organs, while not present in all species of lycaenids, are vital for maintaining many facultative and obligatory relationships with ants, as they secrete nutritious nectar for the ant. These substances are excreted to recruit ants as defenders. Caterpillars secrete generously at first, attracting many ants, and leveling off, once the recruitment is sufficient (Leimar & Axén, 1993). The level of secretion correlates with the level of protection that the caterpillar estimates to require. In studies where there was a simulated predatory attack, the caterpillars exuded more than double the amount of nectar droplets when compared to an undisturbed control (Agrawal & Fordyce, 2000). Furthermore, the caterpillars also induce secretion after the attack as an extra precaution, with the nearly twice the number of secretions following the attack (Leimar & Axén, 1993). This is both favourable for the ants, as an extra reward, but it also allowed the caterpillars to be more protected from a possible following attack. This additional secretion resulted in the ants tending to the caterpillars for an additional 30% of time in the 5 minutes following the attack (Agrawal & Fordyce, 2000). While indirect, the secretion of nectar is the lycaenid’s way of signalling and communicating with the ants. This balance between the secretion reward and ant protection maintains the symbiotic relationship between the ant and lycaenid.
Chemical Composition of Secretion
As is often the case in nature, the composition of the secretion is a precise balance between not consuming excessive resources and nutrients from the caterpillar, while being desirable for the ant. From a study on three species of lycaenids, the nectar is composed primarily of sucrose, an energy-rich disaccharide with smaller amounts of glucose and trace amounts of other sugars (Daniels et al., 2004). Meanwhile, another study found that the sugar in species Niphanda fusca,whose diet is insect-based, was nearly all trehalose (Hojo et al., 2008); trehalose is the dominant sugar in the hemolymph, which is analogous to the blood of insects (Daniels et al., 2004). It is thus inferred that the secretion is directly linked to the diet of the caterpillar (Daniels et al., 2004).
An important similarity between the nectars of differing species is the presence of a dominant amino acid (Daniels et al., 2004). The amino acid plays a key role in the appeal of the sugar. The dominant amino acid in the N. fusca species is glycine (Hojo et al., 2004). When ant species associated with N. fusca and another species that was not were offered pure trehalose and a mixture of trehalose and glycine, the associated ants had a clear preference for the mixture, while the control ants chose both equally (Hojo et al., 2004). Meanwhile, a mixture of glycine and sucrose had no difference between both species, showing that the presence of glycine alone is not what triggers a preference (Hojo et al., 2004). These indicate that the mixture of this specific dominant amino acid and the dominant sugar generates a response in the ants. A preferential feeding response could be due to extraneous exposure, but in the study, both the control and associated ant species had not been previously exposed to the lycaenid nectar, suggesting that this response is innate to the associated ant species (Hojo et al., 2004). The chemical composition of the lycaenid nectar is tailored towards the specific ant species, making the ant feel as if it is receiving a more quality food. This is consistent seeing that the composition of the nectars from two other lycaenid species Polyommatus coridon and Polymmatus icarus is composed of different amino acids, but with one leading, being leucine (Fig. 1) and tyrosine (Fig. 2) respectively (Daniels et al., 2004). Furthermore, species in facultative relationships such as P. coridon and P. icarus, have lower overall levels of amino acids and higher concentrations of other amino acids when compared to those in obligate relationships, such as Jalmenus evagoras (Fig. 3) (Daniels et al., 2004; Pierce & Elgar, 1985).
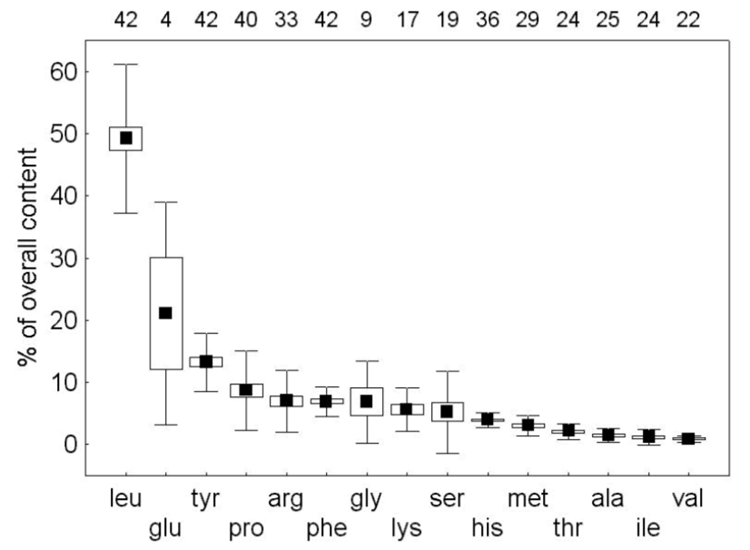
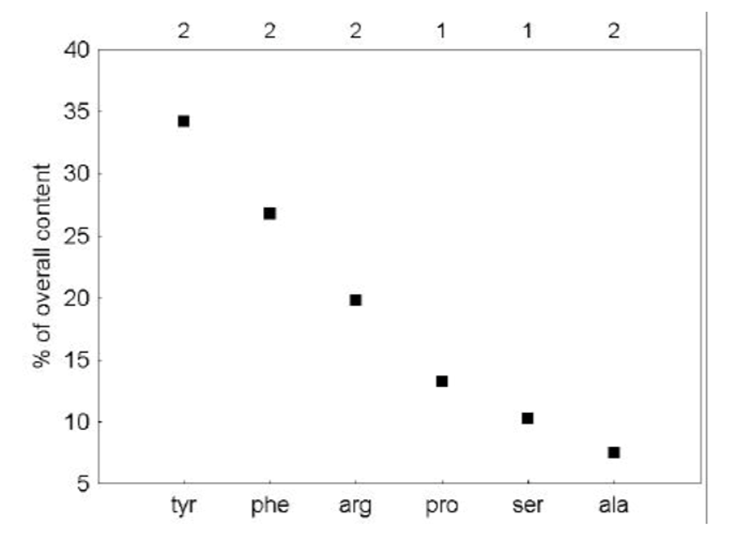
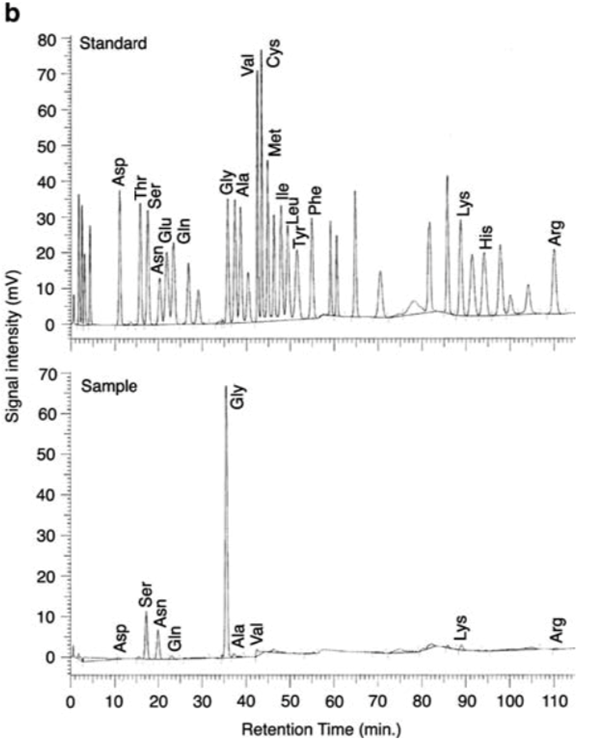
This makes their nectar appeal to a wider range of ant species because the facultative relationship does not bind them to a specific species. The specificity of the composition of lycaenid nectar across species is a clear adaptation of the symbiotic relationship formed between the butterfly and the ant.
The gustatory synergy of certain amino acids and sugars provide insight into manufacturing desirable compounds while being cost-effective. These findings can be used to exploit the taste preferences animals and help engineer solutions to the consumption of otherwise undesirable compounds.
Honey Bees Detect and Avoid Ant Pheromones
One of the most important mutualistic relationships in nature is that of the bee and the flower. Bees pollinate plants and flowers, getting food and nutrients for themselves, and helping the plants reproduce. Unfortunately, due to global warming, habitat loss, and pesticide use, bee populations are slowly dying out (Petruzzello, n.d.). This has thrust bees into the spotlight, and scientists have been researching them and their pollination activities more closely.
A natural problem for any mutualistic relationship is interference from other species. In the case of bees and flowers, there are other animals and insects who feed on nectar, but are not pollinators. One of these species is ants. Ants eat nectar from flowers, but they do not pollinate the flowers. Some ants even release antibodies that kill pollen seed (“Ant Pollination,” n.d.). This reduces the opportunities for bees to pollinate the plants. If there is no nectar, the bee has no incentive to visit that flower, so the plant does not get pollinated. Additionally, some ants attack and kill bees, so the bees will actively try to avoid those flowers (Li et al., 2016). All together, the presence of ants reduces the presence of bees on a plant.
But how do bees know which plants to avoid? They need to get food somewhere, and do not want to waste their time with a plant that has had its nectar eaten by an ant. They work around this by using chemical cues. Ants release pheromones to communicate with each other, and bees have learned to recognize these chemicals, and avoid plants with them (Ballantyne & Willmer, 2012; Li et al., 2014; Sidhu & Rankin, 2016). Multiple studies have been done to better understand this behaviour.
A study by Ballantyne and Willmer (2012) found that bees are not inherently repelled by ant scent marks, but can be trained to avoid them. In their experiment, Bombus terrestris, a type of bumblebee, were kept next to a colony of Lasius niger, a type of ant, to familiarize themselves with their scent. Then, they were given 6 feeders to interact with. 3 were unmarked and contained sucrose solutions, while the other 3 were treated with an ant pheromone and contained water. The bees were moved to a new location with marked and unmarked feeders, all containing a sucrose solution. The feeders and markers were replaced after an hour.
Ballantyne and Willmer (2012) found that the bees did not originally avoid the ant scent (Fig. 4). During the first part of the experiment, the bees visited the marked and unmarked feeders in equal amounts. However, in the second and third part, the bees did visit the unmarked feeders less than the marked ones. This shows that the bees learned to avoid that specific scent, after they started to associate it with no nectar. Avoiding ants is not an innate trait for bees, but rather one learned after having negative interactions with them.
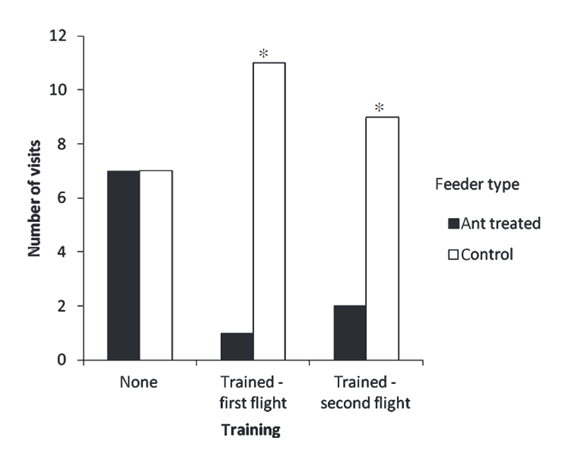
Many other studies have also come to this conclusion, notably Sidhu and Rankin (2016) and Li et al. (2014). Sidhu and Rankin (2016) specifically investigate the behaviour of European honey bees during a drought, while competing with an invasive species of Argentine ants. They found that the bees approached all flowers equally, but accepted flowers without ants at a higher rate than flowers with ants (Fig. 5). In other words, once they were close enough to sense the ant pheromones, they avoided the plant. They found that both the presence of ants, or just the scent of ants, was enough to deter the bees. Even during a drought, when there are limited resources, any sort of mark of an Argentine ant was enough to negatively impact the bee foraging.
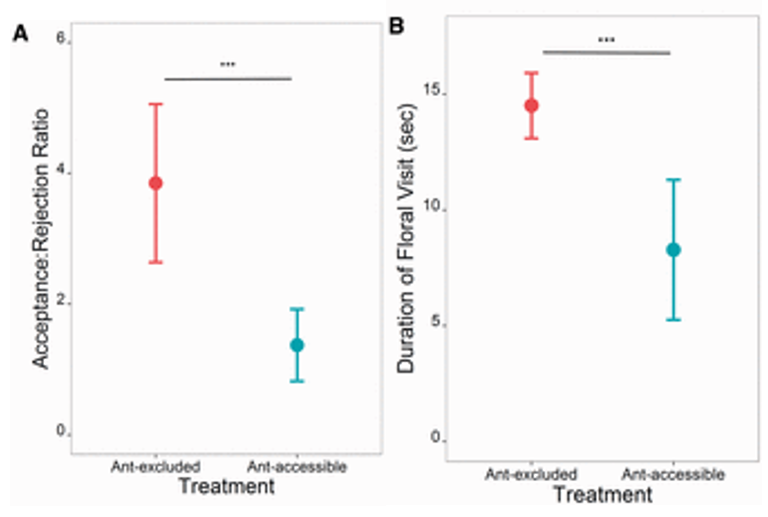
Li et al. (2014) had the most extreme relationship between their studied species. They studied giant Asian honey bees and weaver ants, and weaver ants attack and try to kill honey bees. The bees therefore had a much larger motivation to avoid ants than the previous studies. Unsurprisingly, Li et al. found the same result as the others: bees avoided the flowers with ants, or with ant pheromones. As with Sidhu and Rankin (2016), they found that the bees mainly relied on the olfactory cues. Bees avoided flowers with only pheromones the same amount that they avoided flowers with live ants on them. This is probably because the ants usually hide in the flower, so the bees cannot rely on their sight to avoid them.
Li et al. (2014) also tried to determine the specific chemicals in the ant pheromones the bees were reacting to. Using gas chromatography and mass chromatography, they were able to identify the compounds in the pheromones. They found some compounds in trace amounts, such as nonane, nonanal, and docosane, which they already knew were found in the Dufour gland of the ant, which is the gland that produces the pheromone. They also found that the most abundant compound was heneicosane, which made up over 40% of the pheromone. However, in the experiment, they found that the bees did not avoid heneicosane by itself. This suggests that the bees are either detecting the trace amount of other chemicals there, or the specific mixture of compounds.
But what about the other way? Do ants try to avoid bees? Similarly, if a bee has eaten all the nectar, or if a bee has attacked an ant, ants are not motivated to visit that plant. A study by Phan et al. (2020) found that ants do avoid flowers with bee scent markings. Specifically, they avoided flowers that were marked with honey bee alarm pheromone, honey bee venom sac, honey bee sting bulb, honey bee sting bulb and venom sac, or bumble bee venom sac.
Bees and ants share the same food supply, and therefore must coexist. However, they clearly do not enjoy this, and have learned to avoid each other. Even though they do not do anything to specifically target the other animal, they have each learned the chemical markers related to each other, and know how to react to them.
Chemical Communications for Parasitoid Wasps
Parasitism is an ecological process between two species in which the parasite lives at the host’s expense (Hassell & Waage, 1984). Parasitoid wasps are hymenopteran insects of tiny sizes (Huang et al., 2021). They are born and grown in or on the hosts, known as endoparasitoids and ectoparasitoids respectively (Hassell & Waage, 1984; Huang et al., 2021). Except for searching for enough food to survive, their most vital task is to search for their host insects to lay eggs. They will lay their eggs onto or into the hosts or the hosts’ eggs, which their offspring will kill and use as the source of nutrition (Hassell & Waage, 1984; Huang et al., 2021). Therefore, in agricultural and ecological systems, parasitoid wasps are considered as a type of beneficial insect, which can be used as biological control agents to kill pests, including cerealcrop pests, some pests of vegetable crops, etc. (Hassell & Waage, 1984; Herniou et al., 2013).
However, how can these parasitoid wasps find proper host insects? Chemical communication is the most common way of communication among all organisms, also plays a vitally important role in helping the parasitoid wasps to locate their hosts (Fatouros et al., 2005; J. Matthews & R. Matthews, 2009). Parasitoid wasps can detect and identify some unique volatile chemical compounds and chemical signals from other organisms, including the host insects, other parasitoid wasps, and some plants, even from a long distance (Tumlinson et al., 1993; Turlings et al., 1995; Whitman & Eller, 1990). The complicated interactions of behaviors among parasitoids, hosts, and plants are to a great extent built and mediated chemically (Huang et al., 2021; Tumlinson et al., 1993).
Chemical Communications Between Parasitoid Wasps and Their Hosts
Rather than randomly searching for host insects, parasitoid wasps can use the information from chemical communications with the ability to identify the specific compounds released by their potential hosts to locate them (Hoffmeister & Gienapp, 1999; Tumlinson et al., 1993). For instance, when large cabbage white butterfly Pieris brassicae are mating, a chemical compound, Trichogramma brassicae, will be released by the male butterfly to the female as the anti-aphrodisiac (Fatouros et al., 2005). However, this chemical compound can be easily identified by the parasitoid wasp. When the T. brassicae compound is detected and identified by parasitoid wasps, they will know that the butterfly has mated, and then, they will follow the butterfly by riding on it (Fig. 6) (Fatouros et al., 2005). Thus, they will more easily find their eggs as hosts to parasitize.
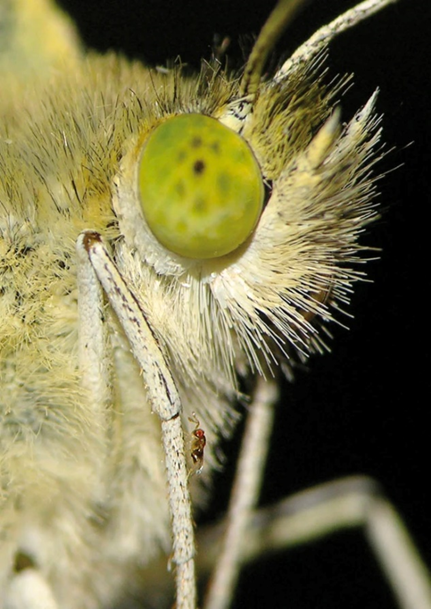
Chemical Communications Amongst Parasitoid Wasps
Parasitoid wasps can also use chemical communications to avoid interfering with each other when oviposition (J. Matthews & R. Matthews, 2009). After finding hosts, they may leave some chemical compounds to show the information they have occupied the hosts for their eggs to their competitors, particularly other parasitoid wasps (J. Matthews & R. Matthews, 2009; Price, 1970). With the information left, other parasitoids will try to find another host for their own eggs instead of still using this host. Therefore, there will be a reduction in competition for both of their offspring with the decrease in population density, and accordingly increase their offspring’s’ survival rate (Price, 1970, 1972). In short, they will form mutualistic relationships, beneficial to each other. The parasitic relationship between a kind of ichneumonid wasp Pleolophus basizonus, and Neodiprion swainei, a native North American sawfly, is a good example (J. Matthews & R. Matthews, 2009). After ovipositing on a sawfly’s egg, the female parasitoid wasps will leave odor trails on the egg (Fig. 7) as pheromone marks (J. Matthews & R. Matthews, 2009). As a result, other parasitoid wasps, even for different kinds of parasitoid wasps other than P. basizonus only, will identify and understand the information given by the pheromone mark. Therefore, they will be significantly less likely to leave their eggs on the hosts occupied already by P. basizonus (J. Matthews & R. Matthews, 2009).
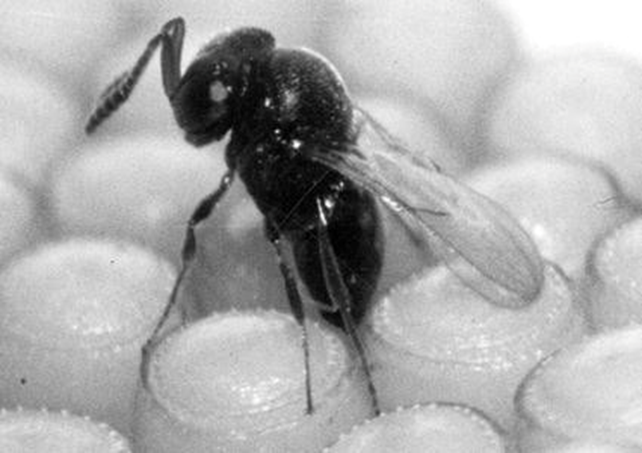
Chemical Communications between Parasitoid Wasps and Plants
Plants and parasitoid wasps can also form mutualism relationship with chemical communications. Some plants may release immune-like chemical compounds when insects attack the plants, in order to attract the attackers’ natural enemies to defend themselves (Tumlinson et al., 1993; Whitman & Eller, 1990). For example, corn seedlings will release chemical signals, several kinds of terpenoids and green leaf volatiles (GLVs), after being attacked by caterpillars (Turlings et al., 1995). The amount of terpenoids and GLVs emission is directly proportional to the areas damaged by the caterpillars (Turlings et al., 1995; Whitman & Eller, 1990). These chemical signals released by the corns are very attractive for parasitoid wasps, who will lay eggs onto or into them as seen in Fig. 8 (Tumlinson et al., 1993; Whitman & Eller, 1990). Consequently, the corn seedlings and the parasitoid wasps form a typical mutualism model. The caterpillars attacked the corns will be killed by the offspring of the parasitoid wasps while the parasitoid wasps also find hosts (Tumlinson et al., 1993).
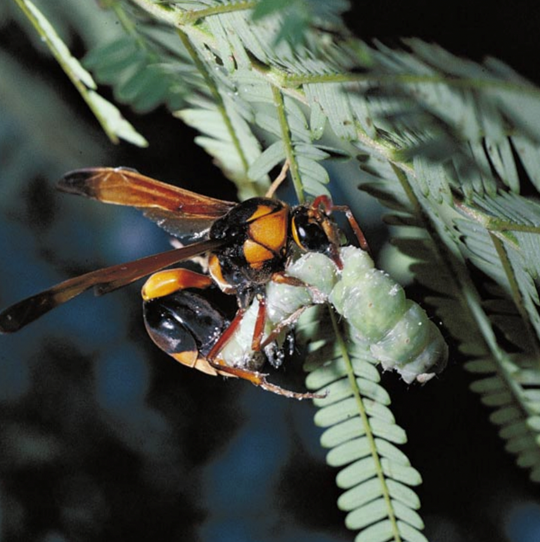
Drosophila-yeast interaction
Sugar-rich sources such as fruits attract fermenting species, such as yeasts. In the presence of oxygen, yeasts convert sugars to ethanol, carbon dioxide, and other metabolic by-products in a sugar-rich place (Cordente et al., 2012). This process is called alcoholic fermentation (Cordente et al., 2012).
To damage and outcompete other competitors, alcoholic fermentation is considered the preferential fermentation for most Saccharomyces yeasts. Indeed, this process helps ATP to be released more quickly (Pfeiffer and Morley, 2014). The high production of ATP leads to a poisonous, hot, and alcoholic environment that kills other competitors (Pfeiffer and Morley, 2014). Yeasts can survive this complex environment because they can catabolize ethanol (Thomson et al., 2005).
Drosophila, a type of fly, are also attracted to sugar-rich fruit. They co-habit with the yeasts. They crowd around fermented food, and they often commit “suicide” by drowning themselves in the fermented foods (Günther & Goddard, 2018). Yeasts play an essential role in the Drosophila diet since these flies feast on yeasts. Research has found that yeasts’ species and available biomass influence Drosophila’s development time and weight (Anagnostou et al., 2010).
The relationship between Drosophila and yeasts seems to be predation, because only the flies benefit from it. To avoid being “eaten” by the animals, the ethanol produced becomes a chemical defense for the yeasts. However, today, fruit flies have evolved, and the ethanol produced can no longer act as an anti-predator adaptation (Günther & Goddard, 2018).
Nonetheless, one strong contrast between these species is that yeasts are immotile and rely upon dispersal facilitated by flies (Günther & Goddard, 2018). Following this key difference, a theory suggests that compounds produced by yeasts act as a signal that attracts fruit flies, and this is helpful as it allows yeast dispersal to new living spaces by flies (Hoang et al., 2015). Assuming this was the situation, the yeast-fly interaction would be bidirectional and take after characters of mutualism (Günther & Goddard, 2018).
In their quest to find the correct theory, many researchers start investigating relationship between Drosophila and yeasts. Christiaens et al. (2014) show in their research that deleting the alcohol acetyltransferase (Atf1) in the yeast cells alters the olfactory response in the antennal lobe of the fruit flies that feast on yeasts (Fig. 9). Being less attracted to yeasts, the flies cannot facilitate the dispersal of mutant yeasts. Therefore, researchers highly believe that acetate esters ethyl acetate and 3-methyl butyl acetate are the two ester volatile compounds produced that attract Drosophila and stimulate yeast dispersal (Christiaens et al., 2014).
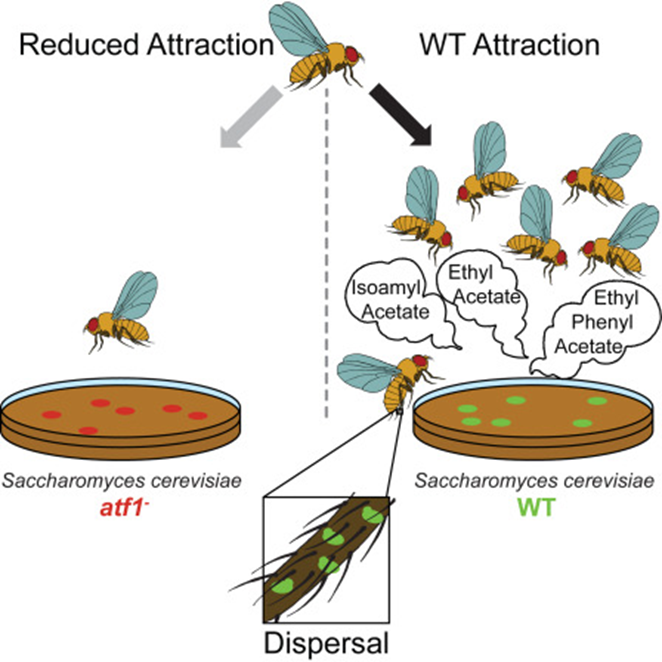
Later, Günther et al. (2019) started by conducting an experiment where two genotypes of Drosophila were exposed to 11 types of yeasts grown in strawberry juice. They measured the Attraction Index (AI) by employing binary tests. As we can see in Figure 10, only one yeast strain (ScNZ) attracted both genotypes of Drosophila. This shows that yeast preference is inconsistent.
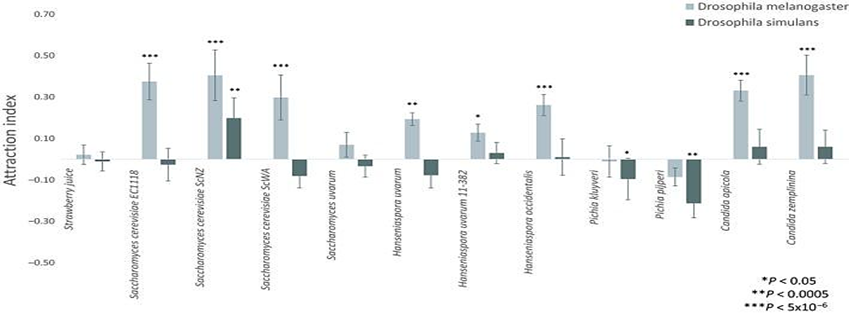
If the “supposed” mutualism between flies and yeasts truly works through chemical communication, then the reaction of different Drosophila genotypes must be consistent with one specific yeast genotype (Günther et al., 2019). However, the experience results reject this general hypothesis, as the response is inconsistent, and only one yeast genotype appears attractive to both genotypes of Drosophila. Also, the data shows that Drosophila simulans are mainly indifferent to the yeasts, whereas D. melanogaster primarily is attracted to yeasts (Günther et al., 2019).
Furthermore, to examine if yeast preference stays unchanged over time, Günther et al. (2019) conducted a second experiment where they exposed 10 generations of Drosophila simulans to two yeast genotypes, specifically the most-attracted yeast (ScNZ) and the least attracted one (ScWA) in strawberry juice. As we can see, the Drosophila reactions are not conserved. In such a short amount of time, D. simulans become attracted to the originally less‐preferable yeast strain (Fig. 11).
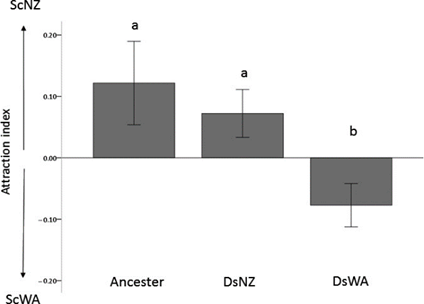
This set-up of trials shows that individual lines of D. melanogaster/D. simulans and Saccharomycetaceae yeasts interactions vary. Further, the Drosophila preference might change over somewhat short biological timescales.
These research examples show that the proof of the mutualism hypothesis enunciated is quite inconsistent (Günther & Goddard, 2018). The experiment by Christiaens et al. (2019) shows that yeast dispersal decreases due to the gene Aft1 absence and does not suggest that volatile yeast production leads to mutualism. Moreover, the yeast preference varies from one genotype to another, and it changes over time; this indicates that no fixed Drosophila-yeast interaction is maintained.
In conclusion, to reject or support the theory of mutualism, we must conduct more experiments on multiple combinations of fly and yeast genotypes. The number of combinations should be large enough to make a general claim. Other than that, we must prove that these interactions are not temporary. Overall, this is a huge task, but having a good understanding of Drosophila is valuable since this fly is commonly used in the experiment due to its characteristics of being cheap and straightforward to cultivate in research center conditions. Bioengineering’s evolution is needed to facilitate further researches on this domain and to reach a general conclusion on the relationship between Drosophila and yeast.
Conclusion
Chemical exchange between species provides a sophisticated and specific means of communication between vastly different species. Such chemical interactions drive the numerous symbiotic and parasitic relationships, with adaptations observed in species to better chemically communicate to increase their survival, whether it is by reducing mutual competition between bees and ants or parasitoid wasps use chemical signals to communicate with other species to search for hosts to lay eggs. Studies of such precise interactions between these small organisms provide insight to engineering more specific communicative devices through chemical pathways, as well as possible chemical triggers, such as gustatory responses, that can be utilized to induce beneficial side effects.
References
Agrawal, A. A., & Fordyce, J. A. (2000). Induced indirect defence in a lycaenid-ant association: the regulation of a resource in a mutualism. Proceedings of the Royal Society of London. Series B: Biological Sciences, 267(1455), 1857-1861. https://doi.org/doi:10.1098/rspb.2000.1221
Akino, T., Knapp, J. J., Thomas, J. A., & Elmes, G. W. (1999). Chemical mimicry and host specificity in the butterfly Maculinea rebeli, a social parasite of Myrmica ant colonies. Proceedings of the Royal Society of London. Series B: Biological Sciences, 266(1427), 1419-1426. https://doi.org/doi:10.1098/rspb.1999.0796
Anagnostou, C., Dorsch, M., & Rohlfs, M. (2010). Influence of dietary yeasts on Drosophila melanogaster life-history traits. Entomologia Experimentalis et Applicata, 136(1), 1-11. https://doi.org/https://doi.org/10.1111/j.1570-7458.2010.00997.x
Ant Pollination. (n.d.). U.S. Forest Service. Retrieved November 5, 2021, from https://www.fs.fed.us/wildflowers/pollinators/animals/ants.shtml
Ballantyne, G., & Willmer, P. (2012). Floral visitors and ant scent marks: noticed but not used? Ecological Entomology, 37(5), 402-409. https://doi.org/https://doi.org/10.1111/j.1365-2311.2012.01378.x
Christiaens, J. F., Franco, L. M., Cools, T. L., De Meester, L., Michiels, J., Wenseleers, T., Hassan, B. A., Yaksi, E., & Verstrepen, K. J. (2014). The fungal aroma gene ATF1 promotes dispersal of yeast cells through insect vectors. Cell Rep, 9(2), 425-432. https://doi.org/10.1016/j.celrep.2014.09.009
Cordente, A. G., Curtin, C. D., Varela, C., & Pretorius, I. S. (2012). Flavour-active wine yeasts. Appl Microbiol Biotechnol, 96(3), 601-618. https://doi.org/10.1007/s00253-012-4370-z
Daniels, H., Gottsberger, G., & Fiedler, K. (2005). Nutrient Composition of Larval Nectar Secretions from Three Species of Myrmecophilous Butterflies. Journal of Chemical Ecology, 31(12), 2805-2821. https://doi.org/10.1007/s10886-005-8395-y
Fatouros, N. E., Huigens, M. E., van Loon, J. J. A., Dicke, M., & Hilker, M. (2005). Butterfly anti-aphrodisiac lures parasitic wasps. Nature, 433(7027), 704-704. https://doi.org/10.1038/433704a
Günther, C., & Goddard, M. (2018). Do yeasts and Drosophila interact just by chance? Fungal Ecology, 38. https://doi.org/10.1016/j.funeco.2018.04.005
Günther, C. S., Knight, S. J., Jones, R., & Goddard, M. R. (2019). Are Drosophila preferences for yeasts stable or contextual? Ecol Evol, 9(14), 8075-8086. https://doi.org/10.1002/ece3.5366
Hassell, M. P., & Waage, J. K. (1984). Host-Parasitoid Population Interactions. Annual Review of Entomology, 29(1), 89-114. https://doi.org/10.1146/annurev.en.29.010184.000513
Herniou, E. A., Huguet, E., Thézé, J., Bézier, A., Periquet, G., & Drezen, J.-M. (2013). When parasitic wasps hijacked viruses: genomic and functional evolution of polydnaviruses. Philosophical Transactions of the Royal Society B: Biological Sciences, 368(1626), 20130051. https://doi.org/doi:10.1098/rstb.2013.0051
Hoang, D., Kopp, A., & Chandler, J. A. (2015). Interactions between Drosophila and its natural yeast symbionts-Is Saccharomyces cerevisiae a good model for studying the fly-yeast relationship? PeerJ, 3, e1116. https://doi.org/10.7717/peerj.1116
Hoffmeister, T. S., & Gienapp, P. (1999). Exploitation of the Host’s Chemical Communication in a Parasitoid Searching for Concealed Host Larvae. Ethology, 105(3), 223-232. https://doi.org/https://doi.org/10.1046/j.1439-0310.1999.00361.x
Hojo, M. K., Wada-Katsumata, A., Ozaki, M., Yamaguchi, S., & Yamaoka, R. (2008). Gustatory synergism in ants mediates a species-specific symbiosis with lycaenid butterflies. Journal of Comparative Physiology A, 194(12), 1043-1052. https://doi.org/10.1007/s00359-008-0375-6
Huang, J., Chen, J., Fang, G., Pang, L., Zhou, S., Zhou, Y., Pan, Z., Zhang, Q., Sheng, Y., Lu, Y., Liu, Z., Zhang, Y., Li, G., Shi, M., Chen, X., & Zhan, S. (2021). Two novel venom proteins underlie divergent parasitic strategies between a generalist and a specialist parasite. Nature Communications, 12(1), 234. https://doi.org/10.1038/s41467-020-20332-8
Leimar, O., & Axén, A. H. (1993). Strategic behaviour in an interspecific mutualism: interactions between lycaenid larvae and ants. Animal Behaviour, 46(6), 1177-1182. https://doi.org/https://doi.org/10.1006/anbe.1993.1307
Li, J., Wang, Z., Tan, K., Qu, Y., & Nieh, J. C. (2014). Giant Asian honeybees use olfactory eavesdropping to detect and avoid ant predators. Animal Behaviour, 97, 69-76. https://doi.org/https://doi.org/10.1016/j.anbehav.2014.08.015
Matthews, R. W., & Matthews, J. R. (2010). Chemical Communication. In Insect Behavior: 2nd Edition (pp. 217-259). Springer Netherlands. https://doi.org/10.1007/978-90-481-2389-6_6
Petruzzello, M. (n.d.). What Would Happen If All the Bees Died? Encyclopedia Britannica. Retrieved November 5, 2021, from https://www.britannica.com/story/what-would-happen-if-all-the-bees-died
Pfeiffer, T., & Morley, A. (2014). An evolutionary perspective on the Crabtree effect [Perspective]. Frontiers in Molecular Biosciences, 1. https://doi.org/10.3389/fmolb.2014.00017
Phan, P. T., Wilson Rankin, E. E., & Purcell, J. (2020). Formica francoeuri responds to pheromones and defensive chemical cues of social bees. Insectes Sociaux, v. 67(no. 4), pp. 547-556-2020 v.2067 no.2024. https://doi.org/10.1007/s00040-020-00788-z
Pierce, N. E., Braby, M. F., Heath, A., Lohman, D. J., Mathew, J., Rand, D. B., & Travassos, M. A. (2002). The Ecology and Evolution of Ant Association in the Lycaenidae (Lepidoptera). Annual Review of Entomology, 47(1), 733-771. https://doi.org/10.1146/annurev.ento.47.091201.145257
Pierce, N. E., & Elgar, M. A. (1985). The influence of ants on host plant selection by Jalmenus evagoras, a myrmecophilous lycaenid butterfly. Behavioral Ecology and Sociobiology, 16(3), 209-222. https://doi.org/10.1007/BF00310983
Price, P. W. (1970). Characteristic Permitting Coexistence Among Parasitoids of a Sawafly in Quebec. Ecology, 51(3), 445-454. https://doi.org/https://doi.org/10.2307/1935379
Price, P. W. (1972). Behavior of the Parasitoidpleolophus basizonus (hymenoptera: Ichneumonidae) in response to changes in host and parasitoid density. The Canadian Entomologist, 104(1), 129-140. https://doi.org/10.4039/Ent104129-1
Sidhu, C. S., & Wilson Rankin, E. E. (2016). Honey Bees Avoiding Ant Harassment at Flowers Using Scent Cues. Environmental Entomology, 45(2), 420-426. https://doi.org/10.1093/ee/nvv230
Thomson, J. M., Gaucher, E. A., Burgan, M. F., De Kee, D. W., Li, T., Aris, J. P., & Benner, S. A. (2005). Resurrecting ancestral alcohol dehydrogenases from yeast. Nat Genet, 37(6), 630-635. https://doi.org/10.1038/ng1553
Tumlinson, J. H., Lewis, W. J., & Vet, L. E. M. (1993). How parasitic wasps find their hosts. Scientific American, 268(3), 100–106. https://doi.org/10.1038/scientificamerican0393-100
Turlings, T. C., Loughrin, J. H., McCall, P. J., Röse, U. S., Lewis, W. J., & Tumlinson, J. H. (1995). How caterpillar-damaged plants protect themselves by attracting parasitic wasps. Proceedings of the National Academy of Sciences, 92(10), 4169-4174. https://doi.org/doi:10.1073/pnas.92.10.4169
Whitman, D. W., & Eller, F. J. (1990). Parasitic wasps orient to green leaf volatiles. Chemoecology, 1(2), 69-76. https://doi.org/10.1007/BF01325231